Molecular profiling of a case of advanced pancreatic cancer identifies an active and tolerable combination of targeted therapy with backbone chemotherapy
Case presentation
A 62-year-old Iranian male without significant past medical history presented with weight loss and painless jaundice in November 2013. A CT scan of the chest, abdomen and pelvis identified multiple liver masses and a presumptive pancreatic mass. He underwent endoscopic retrograde cholangiopancreatography (ERCP) and had a metallic stent placed with marked improvement of his symptoms. CT-guided biopsy of the liver mass was performed and pathology was consistent with moderately differentiated adenocarcinoma, morphologically consistent with metastatic spread from a pancreatic primary (stage IVB).
The patient was started on palliative chemotherapy with gemcitabine and erlotinib by his primary oncologist in February 2014 based on Moore et al. (1). In March 2014, the patient developed severe nausea and vomiting due to cholecystitis and underwent cholecystectomy. Repeat CT scan in April 2014 showed stable disease, however due to persistent severe side effects, gemcitabine and erlotinib were discontinued in mid-May 2014. The patient continued to report progressive fatigue, weight loss and anorexia associated with right upper quadrant and epigastric discomfort. PET/CT 1 month after stopping therapy revealed greater than 20 hypermetabolic liver masses in both lobes, the largest measuring within 4-5 cm, a 5.7 cm × 5 cm pancreatic head mass, and retroperitoneal lymph nodes (Figure 1). Multi-modal molecular profiling of the tumor specimen collected on January 9, 2014 was performed at this time, (Caris Molecular Intelligence®, Irving, TX) and revealed the following alterations detailed in Table 1.
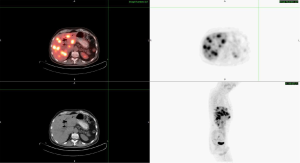
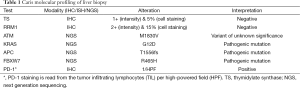
Full table
Interpretation of these findings suggested a likely benefit of capecitabine due to the thymidylate synthase (TS) negativity and or possible response to histone deacetylase inhibitors (HDACi) such as vorinostat based on the ATM mutation. Based on this molecular profile, the treating physician instituted therapy with capecitabine, oxaliplatin and bevacizumab with the addition of vorinostat. After six cycles of therapy, laboratory evaluation revealed improvement in liver function, and marked decline in CEA from 115 to 8 mcg/L and CA 19-9 from 80,000 to 1,525 U/mL. Repeat PET/CT imaging in November 2014 revealed a greater than 50% decrease in the size of the pancreatic head lesion to 2.5 cm × 2.5 cm, with a corresponding decrease in PET avidity. Additionally, there was complete resolution of the previously noted hepatic metastases as well as the retroperitoneal lymphadenopathy (Figure 2). This represents at least an 80-90% response to current therapy by PET/CT.
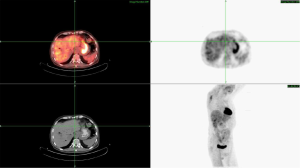
Discussion
Pancreatic adenocarcinoma is the 4th leading cause of cancer mortality in the United States. The disease is particularly deadly, with approximately 82% of those affected ultimately dying of their disease (2). Patients with localized disease who are eligible for surgical resection still have a median survival of only 22.8 months due to high recurrence rates (3). Lack of effective screening tools results in the majority of affected individuals presenting with advanced metastatic disease for which palliative chemotherapy is standard. Standard first line regimens include gemcitabine alone, with erlotinib, or with nab-paclitaxel; or folinic acid, 5-fluorouracil, irinotecan, and oxaliplatin (FOLFIRINOX). Currently, median survival with single agent gemcitabine is 5-7 months compared to 8.5-11 months with newer chemotherapeutic regimens such as FOLFIRINOX and gem/nab-paclitaxel (4,5). There are no standard therapies after failure or intolerance of 1st line therapy. Although FOLFIRINOX and gem/nab-paclitaxel have produced an improved overall survival, they are best applied to a patient population with good performance status due to their association with increased toxicities. To date, targeted therapy in pancreatic cancer has been limited to erlotinib, a small-molecule tyrosine-kinase inhibitor of EGFR used in combination with gemcitabine. However, this combination therapy has only resulted in a very subtle median overall survival advantage measured in days (1). Therefore, well-designed clinical trials to identify impactful targeted therapy in pancreatic adenocarcinoma are desperately needed.
Over recent years therapy in oncology has moved away from traditional chemotherapy due to the identification of “drug-able” targets in multiple solid malignancies. For instance, in advanced melanoma 40% of patients have been found to have a BRAF V600E mutation that allows for the use of BRAF inhibitors such as vemurafenib and dabrafenib as well as MEK inhibitors such as trametinib, which both alone and in combination have demonstrated improved survival in tumors with these mutations (6,7). This same success was reached in advanced non-small cell lung cancer (NSCLC), as identification of patients with an EML4-ALK translocation allows for the use of the small molecule tyrosine kinase inhibitor crizotinib which nearly doubles median overall survival when compared to standard doublet chemotherapy (8). Likewise, activating EGFR mutations have shown dramatic responses to anti-EGFR tyrosine kinase inhibitors such as erlotinib and afatinib (9).
To that end, potential actionable targets in pancreatic cancer are actively being investigated. Recently a review of the Catalogue of Somatic Mutations in Cancer (COSMIC) (10) database (accessed December 1, 2014) reveals that most pancreatic cancers contain somatic mutations, the most common of which include KRAS, TP53, CDKN2A, SMAD4, and ARID1A. Other mutations involved include ATM, FBXW7, and APC which were present in our patient as well as MLL3, PIK3CA, BRAF and STK11/LKB1. Early use of next generation sequencing (NGS) in 24 pancreatic cancers revealed an average of 63 mutations and 12 core signaling pathways of which at least one, and usually many, is genetically altered in the majority of tumors analyzed (11). Of note, key pathways identified include apoptosis, DNA damage control, regulation of G1/S phase, hedgehog, homophilic cell adhesion, integrin signaling, c-Jun N-terminal, Wnt/Notch, KRAS, regulation of invasion, small GTPase-dependent signaling, and TGF-β.
KRAS or V-Ki-ras2 Kirsten rat sarcoma viral oncogene homolog encodes a signaling intermediate involved in many signaling cascades including the EGFR pathway. Greater than seventy percent of pancreatic cancer samples in the COSMIC cohort contain a KRAS mutation (12). KRAS regulates cell survival and differentiation by activating multiple signaling pathways. Mutations in KRAS interfere with the hydrolysis of GTP resulting in a constitutively active state (13). These mutations are common in pancreatic ductal cancer cells and play a significant role in the early development of malignancy (14).
KRAS somatic mutations have been found in pancreatic (57%), colon (35%), lung (16%), biliary tract (28%), and endometrial (15%) cancers. Mutations at activating hotspots are associated with resistance to EGFR tyrosine kinase inhibitors (erlotinib, gefitinib) in NSCLC and monoclonal antibodies (cetuximab, panitumumab) in CRC patients. Current clinical trials are focusing on key downstream targets of KRAS, such as MEK, for which multiple inhibitors are in development. To date, MEK inhibition in pancreatic cancer has not resulted in major gains. A randomized phase II trial in pancreatic cancer with the MEK inhibitor trametinib in combination with gemcitabine versus gemcitabine alone did not result in a statistically significant prolongation of overall survival, despite achieving a 22% response rate in the combination arm (15). Combining MEK inhibitors with other agents in pancreatic cancer remains a focus of clinical investigation. Of note, our patient was unable to tolerate erlotinib therapy and did not achieve a measurable response.
APC or adenomatous polyposis coli are a key tumor suppressor gene that encodes for a large multi-domain protein (16). This protein exerts its tumor suppressor function in the Wnt/β-catenin cascade mainly by controlling the degradation of β-catenin, the central activator of transcription in the Wnt signaling pathway. The Wnt signaling pathway mediates important cellular functions including intercellular adhesion, stabilization of the cytoskeleton, and cell cycle regulation and apoptosis, and it is important in embryonic development and oncogenesis. Mutation in APC results in a truncated protein product with abnormal function, lacking the domains involved in β-catenin degradation. Somatic mutation in the APC gene can be detected in the majority of colorectal tumors (80%) and it is an early event in colorectal tumorigenesis. APC wild type patients have shown better disease control rate in the metastatic setting when treated with oxaliplatin, while when treated with fluoropyrimidine regimens, APC wild type patients experience more hematological toxicities. APC mutation has also been identified in oral squamous cell carcinoma, gastric cancer as well as hepatoblastoma and may contribute to cancer formation. Various clinical trials (on www.clinicaltrials.gov) investigating agents which target this gene and/or its downstream or upstream effectors maybe available for APC mutated patients. Our patient was in fact APC mutated suggesting a diminished response to oxaliplatin alone, however a more hematologic tolerability to the addition of 5-FU based therapies.
The identification of biomarkers to predict chemotherapeutic sensitivity has been extensively studied. Of note, the use of TS represents a classic example of this pursuit. TS is a crucial enzyme that is involved in one of the nucleotide biosynthesis pathways and converts deoxyuridine monophosphate (dUMP) to deoxythymidine monophosphate (dTMP) through methylation via 5,10-methylene tetrahydrofolate [CH(2)THF] (17). As we know, 5-FU, an antimetabolite, is one of the most frequently used anti-neoplastic agents in cancer therapy today. Once 5-FU enters the cell it is metabolized into 5-fluorodeoxyuridine monophosphate (FdUMP). FdUMP produces a stable enzyme complex with TS and CH(2)THF resulting in significant inhibition of the thymine biosynthesis pathway, depleting the nucleotide dTTP (18,19), thereby abrogating DNA replication. Of note, TS expression markedly varies in human malignancies (20,21). Therefore, the therapeutic response to 5-FU may have a wide range of clinical variability based on the expression levels of TS. To date the literature regarding whether TS expression accurately predicts for 5-FU or other fluoropyrimidines response remains inconsistent (22). However, in our case, TS expression was negative and our patient had a significant response to a capecitabine-containing backbone therapy.
ATM or ataxia telangiectasia mutated is activated by DNA double-strand breaks and DNA replication stress. It encodes a protein kinase that acts as a tumor suppressor and regulates various biomarkers involved in DNA repair, which include p53, BRCA1, CHK2, RAD17, RAD9, and NBS1. Although ATM is associated with hematologic malignancies, somatic mutations have been found in colon (18%), head and neck (14%), and prostate (12%) cancers. Inactivating ATM mutations make patients potentially more susceptible to PARP inhibitors. Of note, the ATM gene controls aspects of both DNA repair and multiple cell cycle checkpoints (23). Therefore, in cells with mutated ATM, both DNA repair and cell cycle control pathways are faulty. FBXW7 is a gene involved in the regulation of G1/S phase transition regulating a pathway that is commonly mutated in pancreatic cancer. Wild type FBXW7 is involved in ubiquitin-mediated degradation of oncoproteins. Low levels have been found to be associated with poorer survival and increased resistance to chemotherapy, specifically taxane-based. In NSCLC cell lines, when FBXW7 is silenced, the sensitivity to taxane therapy returns with treatment using an HDACi (24). Histone acetylation/deacetylation modifies the state of chromatin domains and thereby affects gene transcription, a process regulated by HDACi. In addition to their role in histone modification, HDACi have also been shown to impact activation of DNA repair. In cells with mutated ATM as in our patient, it is reasonable to assume that the application of HDACi could further compromise the DNA repair pathway, resulting in the cell initiating an apoptotic response (25).
HDACi and fluoropyrimidines are synergistic as well as known radiation sensitizers (26). An ongoing phase I clinical trial evaluating the use of combination therapy with capecitabine, HDACi vorinostat, and external-beam radiation therapy (RT) in patients with non-metastatic pancreatic cancer has reported preliminary results supporting activity of the combination (27). Diffusion weighted-MRIs were obtained pre treatment and 1 week after treatment to evaluate tumor cellularity. Peripheral blood mononuclear cells (PBMCs) were also collected pre treatment, during treatment and after completion of treatment to assess HDAC activity. The 18 patients enrolled on the study included resectable (n=1); borderline resectable (n=9); and unresectable (n=8). Common adverse events were lymphopenia, GI toxicity, and fatigue. HDACi and apparent diffusion coefficient decrease on DWI-MRI was identified. This regimen was found to be a tolerable with very minimal toxicity. The trial is ongoing with accrual to the final cohort of vorinostat at 400 mg (26). Though results have not been reported, the fact that the final planned dose cohort was reached may suggest that the combination of fluoropyrimidines and HDACi thus far represents a tolerable regimen in non-metastatic pancreatic cancer.
Further investigation is warranted to implore the use of HDACi with backbone chemotherapy in metastatic pancreatic cancer dependent upon a patient’s specific mutational profile, considering its acceptable tolerability and impressive response to therapy. Recent clinical trials that have been concluded in pancreatic cancer include a combination of proteasome inhibitors and HDACi, HDACi plus RT, and HDACi plus RT and infusional 5-FU. A trial of gemcitabine, nab-paclitaxel, sorafenib and vorinostat in previously untreated stage IA, IB, IIA, IIB and III pancreatic cancer patients is now actively recruiting (www.clinicaltrials.gov, NCT02349867).
VEGF and its receptors are frequently overexpressed in pancreatic cancer and other adenocarcinomas. CALGB 80303 was a randomized trial comparing gemcitabine + bevacizumab vs. gemcitabine alone with a primary end point of overall survival. The trial was not statistically significant but PFS was marginally better (P=0.07) (28). A second randomized trial evaluated gemcitabine + erlotinib + bevacizumab vs. gemcitabine + erlotinib (29). The primary endpoint was OS which was not statistically significant however the secondary end point of PFS was significantly better (P=0.0002; HR =0.73). Although bevacizumab is not standard of care these findings suggest some value in its use as a therapeutic option for metastatic pancreatic cancer. Our case highlights the possibility of a level of clinically relevant synergism between HDACi and anti-angiogenesis warranting further investigation of this combination therapy based on NGS.
Of note, our case also reported PD-1 positivity in the patient’s mutational profile. While PD-1 is not a known biomarker for the activity of any molecules, it may serve as an indicator that the tumor specimen has some degree of immunogenicity, which could speak to a potential role for immunotherapy in this disease.
Conclusions
As of 2015, the overall prognosis of pancreatic cancer remains grave with a five year overall survival less than 5% (30). For metastatic pancreatic cancer, systemic chemotherapy with FOLFIRINOX or gemcitabine and nab-paclitaxel offer patients the best median over survival. In other solid malignancies such as melanoma and NSCLC, the advent of targeted therapy has resulted in significant progress in terms of overall outcomes for patients whose tumors harbor specific mutations or alterations. Pancreatic cancer has multiple potentially “drug-able” targets as defined in both the COSMIC database as well as NGS of pancreatic tumor tissue. Interestingly, to date <10% of clinical trials in pancreatic cancer involve the identification and use of a biomarker of significance. Our case highlights that the identification of multiple mutational targets allows for a personally tailored focus in regards to therapy. For the first time we report that the addition of an HDACi and an anti-VEGF agent to acceptable backbone chemotherapy of CapeOx (31) for metastatic pancreatic cancer results in significant overall response rate. Further investigation utilizing this targeted approach with backbone chemotherapy in large scale clinical trials is warranted. Considering the numbers of somatic mutations generally identified in most pancreatic cancers, focusing on a single mutational target alone is unlikely to result in significant clinical impact.
Acknowledgements
None.
Footnote
Conflict of Interest: The authors have no conflicts of interest to declare.
References
- Moore MJ, Goldstein D, Hamm J, et al. Erlotinib plus gemcitabine compared with gemcitabine alone in patients with advanced pancreatic cancer: a phase III trial of the National Cancer Institute of Canada Clinical Trials Group. J Clin Oncol 2007;25:1960-6. [PubMed]
- Siegel RL, Miller KD, Jemal A. Cancer statistics, 2015. CA Cancer J Clin 2015;65:5-29. [PubMed]
- Oettle H, Neuhaus P, Hochhaus A, et al. Adjuvant chemotherapy with gemcitabine and long-term outcomes among patients with resected pancreatic cancer: the CONKO-001 randomized trial. JAMA 2013;310:1473-81. [PubMed]
- Von Hoff DD, Ervin T, Arena FP, et al. Increased survival in pancreatic cancer with nab-paclitaxel plus gemcitabine. N Engl J Med 2013;369:1691-703. [PubMed]
- Conroy T, Desseigne F, Ychou M, et al. FOLFIRINOX versus gemcitabine for metastatic pancreatic cancer. N Engl J Med 2011;364:1817-25. [PubMed]
- Chapman PB, Hauschild A, Robert C, et al. Updated overall survival (OS) results for BRIM-3, a phase III randomized, open-label, multicenter trial comparing BRAF inhibitor vemurafenib (vem) with dacarbazine (DTIC) in previously untreated patients with BRAFV600E-mutated melanoma. J Clin Oncol 2012;30:abstr 8502.
- Flaherty KT, Infante JR, Daud A, et al. Combined BRAF and MEK inhibition in melanoma with BRAF V600 mutations. N Engl J Med 2012;367:1694-703. [PubMed]
- Shaw AT, Kim DW, Nakagawa K, et al. Crizotinib versus chemotherapy in advanced ALK-positive lung cancer. N Engl J Med 2013;368:2385-94. [PubMed]
- Zhou C, Wu YL, Chen G, et al. Erlotinib versus chemotherapy as first-line treatment for patients with advanced EGFR mutation-positive non-small-cell lung cancer (OPTIMAL, CTONG-0802): a multicentre, open-label, randomised, phase 3 study. Lancet Oncol 2011;12:735-42. [PubMed]
- Heestand GM, Kurzrock R. Molecular landscape of pancreatic cancer: implications for current clinical trials. Oncotarget 2015;6:4553-61. [PubMed]
- Jones S, Zhang X, Parsons DW, et al. Core signaling pathways in human pancreatic cancers revealed by global genomic analyses. Science 2008;321:1801-6. [PubMed]
- Forbes SA, Bindal N, Bamford S, et al. COSMIC: mining complete cancer genomes in the Catalogue of Somatic Mutations in Cancer. Nucleic Acids Res 2011;39:D945-50. [PubMed]
- di Magliano MP, Logsdon CD. Roles for KRAS in pancreatic tumor development and progression. Gastroenterology 2013;144:1220-9. [PubMed]
- Hruban RH, Goggins M, Parsons J, et al. Progression model for pancreatic cancer. Clin Cancer Res 2000;6:2969-72. [PubMed]
- Infante JR, Somer BG, Park JO, et al. A randomised, double-blind, placebo-controlled trial of trametinib, an oral MEK inhibitor, in combination with gemcitabine for patients with untreated metastatic adenocarcinoma of the pancreas. Eur J Cancer 2014;50:2072-81. [PubMed]
- Fearnhead NS, Britton MP, Bodmer WF. The ABC of APC. Hum Mol Genet 2001;10:721-33. [PubMed]
- Schiffer CA, Clifton IJ, Davisson VJ, et al. Crystal structure of human thymidylate synthase: a structural mechanism for guiding substrates into the active site. Biochemistry 1995;34:16279-87. [PubMed]
- Newman EM, Lu Y, Kashani-Sabet M, et al. Mechanisms of cross-resistance to methotrexate and 5-fluorouracil in an A2780 human ovarian carcinoma cell subline resistant to cisplatin. Biochem Pharmacol 1988;37:443-7. [PubMed]
- Yoshioka A, Tanaka S, Hiraoka O, et al. Deoxyribonucleoside triphosphate imbalance. 5-Fluorodeoxyuridine-induced DNA double strand breaks in mouse FM3A cells and the mechanism of cell death. J Biol Chem 1987;262:8235-41. [PubMed]
- Ciaparrone M, Quirino M, Schinzari G, et al. Predictive role of thymidylate synthase, dihydropyrimidine dehydrogenase and thymidine phosphorylase expression in colorectal cancer patients receiving adjuvant 5-fluorouracil. Oncology 2006;70:366-77. [PubMed]
- Grem JL, Danenberg KD, Behan K, et al. Thymidine kinase, thymidylate synthase, and dihydropyrimidine dehydrogenase profiles of cell lines of the National Cancer Institute's Anticancer Drug Screen. Clin Cancer Res 2001;7:999-1009. [PubMed]
- Koopman M, Venderbosch S, Nagtegaal ID, et al. A review on the use of molecular markers of cytotoxic therapy for colorectal cancer, what have we learned? Eur J Cancer 2009;45:1935-49. [PubMed]
- Thurn KT, Thomas S, Raha P, et al. Histone deacetylase regulation of ATM-mediated DNA damage signaling. Mol Cancer Ther 2013;12:2078-87. [PubMed]
- Yokobori T, Yokoyama Y, Mogi A, et al. FBXW7 mediates chemotherapeutic sensitivity and prognosis in NSCLCs. Mol Cancer Res 2014;12:32-7. [PubMed]
- Pearl LH, Schierz AC, Ward SE, et al. Therapeutic opportunities within the DNA damage response. Nat Rev Cancer 2015;15:166-80. [PubMed]
- Santini V, Gozzini A, Ferrari G. Histone deacetylase inhibitors: molecular and biological activity as a premise to clinical application. Curr Drug Metab 2007;8:383-93. [PubMed]
- Chan E, Arlinghaus LR, Cardin DB, et al. Phase I trial of chemoradiation with capecitabine and vorinostat in pancreatic cancer. J Clin Oncol 2013;31:abstr 225.
- Kindler HL, Niedzwiecki D, Hollis D, et al. Gemcitabine plus bevacizumab compared with gemcitabine plus placebo in patients with advanced pancreatic cancer: phase III trial of the Cancer and Leukemia Group B (CALGB 80303). J Clin Oncol 2010;28:3617-22. [PubMed]
- Van Cutsem E, Vervenne WL, Bennouna J, et al. Phase III trial of bevacizumab in combination with gemcitabine and erlotinib in patients with metastatic pancreatic cancer. J Clin Oncol 2009;27:2231-7. [PubMed]
- Solomona T, Racheta B, Whitehead S, et al. Cancer Survival in England: Patients diagnosed 2007-2011 and followed up to 2012. Available online: http://www.ons.gov.uk/ons/dcp171778_333318.pdf
- Xiong HQ, Varadhachary GR, Blais JC, et al. Phase 2 trial of oxaliplatin plus capecitabine (XELOX) as second-line therapy for patients with advanced pancreatic cancer. Cancer 2008;113:2046-52. [PubMed]