Immunomodulation in hepatocellular cancer
Introduction
Hepatocellular carcinoma (HCC) is a life threatening malignancy that has become a global healthcare problem (1). HCC is common in regions where hepatitis B and hepatitis C infection are endemic. Alcoholic cirrhosis, diabetes mellitus, obesity, and nonalcoholic steatohepatitis (NASH) are also known risk factors for the development of HCC (2). HCC lethality is multifactorial; several factors are advanced stage at diagnosis, poor residual liver reserve, high rate of recurrence after curative therapies and resistance to chemotherapy. Historically in the United States, HCC is a rare cancer, however its incidence has begun to rise. This may be related to the growing number of hepatitis C carriers and rising frequency of metabolic syndrome. The incidence of HCC has almost tripled since the early 1980s, and it is currently the fastest rising cause of cancer-related deaths in the United States (3,4).
The treatment of HCC is multidisciplinary, involving surgical resection, locoregional therapies, and systemic treatment. For patients with advanced HCC, systemic treatment is the only available option. However, majority of patients unfortunately present with advanced disease with severe liver dysfunction and a poor performance status. Systemic chemotherapeutics are often poorly tolerated with adverse health effects. Particularly, patients with advanced HCC and liver cirrhosis have been shown to have no survival benefit following traditional cytotoxic chemotherapy with poor tolerance (5). Many patients with HCC at the time of diagnosis also have chronic hepatitis infection. Intensive chemotherapy with immunosuppression increases the risk of reactivating a dormant hepatitis infection leading to treatment complications (6). Due to these comorbidities there has been no established chemotherapeutic regimen approved for the treatment of HCC (7). Systemic therapy is therefore limited to sorafenib for the treatment of advanced HCC (8).
Immunosuppressive networks in HCC
Tumor associated antigens (TAAs) are presented on MHC molecules and induce an inflammatory immune response. TAAs include alpha-fetoprotein (AFP), glypican-3 (GPC-3), and cancer/testis proteins (9,10). In addition to TAAs, neo-antigens arising from specific gene mutations can also elicit anti-tumor immune responses. Stimulatory and inhibitory signals orchestrate the optimal activation and maintenance of the immune system. For the priming and activation of effector T-cells (Teffs), the specific interaction of T-cell receptors and TAAs on the MHC molecules, as well as co-stimulation via ligand/receptor complexes between antigen presenting cells (CD28, CD137, and CD27) and T cells (CD80 and CD86, CD137L, and CD70) are required. Immune checkpoint inhibition is the main signaling pathway preventing over-activation of the immune system when immune cells are chronically exposed to infection or malignancy. Tumor cells are able to modulate the immune checkpoint pathway, allowing them to go undetected by the native immune system. Several key molecules of immune checkpoints have been identified, including cytotoxic T-lymphocyte associated protein 4 (CTLA-4), programmed death 1 (PD-1), programmed death ligand 1 (PD-L1), T cell membrane protein 3 (TIM-3), killer cell immunoglobulin-like receptors (KIR), and lymphocyte-activation gene 3 (LAG-3) (11).
HCC evades the anti-tumor immunity by creating a complex immunosuppressive network. HCC cells interact with immune and stromal cells through the secretion of immunosuppressive cytokines (9). HCC derived cytokines are able to reduce immune responses as well as modulates its own growth. Through the EGFR ligand, marrow-derived mesenchymal stem cells promote tumor angiogenesis and fibrogenesis. Complimentary growth factor pathways including transforming growth factor (TGF)-β, vascular endothelial growth factor (VEGF), and platelet-derived growth factor (PDGF) further mediate this response, also enable unregulated tumor growth.
The liver has tolerogenicity, which is believed to be intrinsic to its anatomy and function. As the major route for processing absorbed nutrients and molecules from the gastrointestinal tract, the liver has adapted to minimize its immune response to intestinal bacteria or pathogen-derived molecules (12,13). Cells native to the liver including hepatocytes, liver sinusoidal endothelial cells (LSECs), Kupffer cells, and liver dendritic cells contribute to tolerogenicity by inducing an anergic phenotype in cytotoxic CD8+ T cells (14-16). Under chronic inflammation from HBV or HCV infection, tumor-infiltrating lymphocytes, Kupffer cells or LSECs have an elevated level of PD-1, CTLA-4, and TIM-3 (17-21), leading to immune system exhaustion. Liver dendritic cells are also less immunogenic than those in other organs and have a reduced role as antigen presenting cells (22). Tumor cells secrete immunosuppressive cytokines including IL-8 and IL-10, which directly suppress cytotoxic T-cells and NK cells (23-26). IL-10 is also a known activator of tumor-associated macrophages (TAMs) via the M2 macrophage polarization pathway, and TAMs are known to promote tumor progression and are associated with a poor prognosis (26). Chronic inflammation of HBV and HCV leads to an even further increase in immunosuppressive cytokines including IL-4, IL-5, IL-8, IL-10, and TGF-β (27,28). This interplay of dysregulation of cytokines and upregulation of immune checkpoint-associated molecules in the liver signifies the exhaustion of Teffs and cytotoxic CD8+ T cells (29). The liver’s immune environment in combination with HCC’s immunosuppressive nature is a formidable barrier in the treatment of HCC.
Immunomodulation in HCC
Sorafenib
Sorafenib inhibits tumor-cell proliferation and angiogenesis by blocking both the intracellular Raf kinase pathway and extracellular VEGFRs and PDGFR-β associated kinases. Sorafenib improves survival in patients with advanced HCC (8,30). Patients with HCC and liver cirrhosis were able to tolerate sorafenib with a minimal side effect profile HCC (31). Sorafenib has also been reported to have immunomodulatory effects in addition to enhancing the tyrosine kinase inhibition of cancer proliferation and angiogenesis (32,33). Two studies have shown that sorafenib modulates the tumor microenvironment in a murine mouse model (34,35). In the microenvironment of human HCC, sorafenib enhanced antitumor immunity by relieving intrinsic inhibitions of Teffs (36), modulating dendritic cells (37) and natural killer cells (38). Sorafenib was shown to improve immune responsiveness by increasing the ratio of Teffs to regulatory T cells (Tregs) (39), resulting in reduced expression of immunosuppressive cytokines and growth factors. Sorafenib also enhanced migration of cytotoxic CD8+ T cells and suppressed infiltration of myeloid derived suppressive cells (MDSCs) and Tregs to the liver (32).
Our group demonstrated for the first time that the ratio of CD4+CD127+PD-1− Teffs to CD4+Foxp3+PD-1+ Tregs was significantly increased following treatment with sorafenib in patients with advanced HCC (40). In an in vitro experiment with unsorted T cells, there was a marked reduction in the frequency of CD4+CD127+PD-1+ T cells, whereas the frequency of CD4+CD127+PD-1– T cells was enhanced by sorafenib treatment. Sorafenib also decreased the levels of immunosuppressive cytokines IL-10 and TGF-β1, thereby reducing fibrogenesis and the remodeling of the HCC tumor microenvironment. This study suggests that sorafenib has a direct immunomodulatory effect on lymphocytes, as well as indirect effect on the HCC tumor microenvironment helping to reduce the immunosuppressive network in HCC.
The direct immunomodulatory effects of sorafenib in addition to its tyrosine kinase inhibition suggest that it could be an adjunct in combination with other immunotherapeutic approaches (40,41).
Immune checkpoint inhibitor
Checkpoint inhibitors have significantly expanded the treatment options in a number of solid and hematologic malignancies. Although there are many potential immune checkpoints, CTLA-4 and PD-1 are two main immune checkpoints that have been extensively studied with targeted therapies. CTLA-4 and PD-1 systems help prevent overstimulation of immune responses to both foreign and self-antigens (42-44). CTLA-4 expression is regulated by negative feedback. Increasing activation of T-cell receptors and proinflammatory cytokines results in increased CTLA-4 expression and a muted immune response. PD-1 is a surface molecule expressed on many immune cells including T cells and B cells. Ligands of PD-1 (PD-L1 and PD-L2) are expressed on various tissues including cancer cell surfaces. The specific binding of PD-1 and PD-L1 or PD-L2 leads to immune system exhaustion and upregulation of Tregs (45). As in CTLA-4 expression, the expression of PD-1 and PD-L1 and 2 is also increased by the degree of pro-inflammatory cytokines (46,47). Many side effects from immune checkpoint inhibitors have been reported, but these side effects are often mitigated by anti-inflammatory medications such as glucocorticoids (48-50). Secondary to their immunomodulatory nature, this drug class has been approved for a wide variety of malignancies.
Despite their use in a large number of conditions, few clinical trials have studied the use of checkpoint inhibitors for patients with hepatocellular carcinoma. Sangro et al. (51), performed a phase I clinical trial and reported that tremelimumab, an anti-CTLA-4 antibody showed a partial response rate of 17.6% and disease control rate of 76.4%. Sangro et al. also showed a significant decrease in hepatitis C viral load in patients with inoperable hepatocellular carcinoma and chronic hepatitis C infection. A preliminary report of CheckMate 040 (Phase I/II trial for patients having advanced HCC, including those with hepatitis C virus (HCV), hepatitis B virus (HBV), and uninfected patients) was presented by El-Khoueiry et al. in 2015. It demonstrating that, among 39 patients with advanced hepatocellular carcinoma, 5% and 18% of patients showed complete and partial responses, and overall survival at 6 months was 72% (52). Another preliminary result from CheckMate 040 was reported in January 2017 at the Gastrointestinal Cancers Symposium, American Society of Clinical Oncology (53). Out of 37 patients in the escalation cohort and 145 patients in the expansion cohort, the objective response rates were 16.2% and 18.6%, with a median overall survival of 15.0 and 13.2 months, respectively. PD-L1 expression did not correlate with the response rate to nivolumab. Currently, CheckMate 459 is recruiting patients with advanced hepatocellular carcinoma, comparing nivolumab to sorafenib as a primary treatment (54). These reports on immune-checkpoint inhibitors for patients with advanced hepatocellular carcinoma suggest that nivolumab is well tolerated without many of the side effects reported in patients treated for other malignancies. Nivolumab also had a durable response in patients irrespective of hepatitis B or C viral status comparing nivolumab to sorafenib as a primary treatment (55). Our group will begin a phase II clinical trial with pembrolizumab and sorafenib for patients with advanced HCC in 2017. Table 1 shows clinical trials currently available with checkpoint inhibitors in patients with hepatocellular carcinoma.
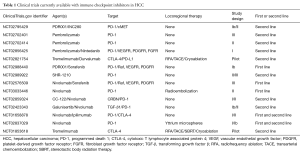
Full table
Tumor ablation and embolization
Locoregional therapies remain a standard of care in HCC although these therapies are not curative for patient with the Barcelona Clinic Liver Cancer (BCLC) intermediate stage (B). Previously, tumor ablation or embolization were considered to locally destroy HCC tumors and slow tumor progression. However, many recent studies have shown that it can also stimulate or modulate tumor immunity.
Radiofrequency ablation (RFA) and microwave ablation (MWA) are the two most common ways to deliver both targeted and large amounts of energy to a tumor. Both of these methods directly induce tumor necrosis by supplying high temperatures and energy. Tumor destruction leads to the release of intracellular components including heat shock proteins, which are highly immunogenic (56). This activates antigen-presenting cells and enhances local tumor immunity (57). Studies have also shown that MWA and RFA change the level of cytokine levels that are directly associated with tumor immunity (58). Patients with higher levels of heat shock proteins in the peripheral blood after treatment have been shown to have improved survival (59,60), and it supports the immunomodulatory effect of RFA and MWA. Ito et al. (61), demonstrated that RFA was significantly immunogenic in a preclinical mouse model when performed prior to surgical resection (pre-resectional RFA). RFA caused a marked increase in antigen-specific CD8+ T cells within the tumor microenvironment and tumor-draining lymph node. It also delayed growth of distant tumors through systemic CD8+ T cell-mediated antitumor immunity.
Other studies have shown that cryoablation can also induce immune-mediated alteration of tumor immunity. While RFA and MWA induce immunogenicity by destroying tumors and releasing cellular debris, cryoablation causes cellular inflammation through low temperatures and freezing tumor cells (62,63). Unlike high energy ablation, cellular components in frozen cells are not destroyed. These intact cellular components, when released, can elicit an immune response (64,65) similar to the necrotic debris in RFA and MWA.
Transarterial chemoembolization (TACE) is used for HCC patients with unresectable, encapsulated tumors, and preserved liver function (66,67). TACE also has a potentially immunomodulatory effect similar to RFA or MWA. TACE has been shown to increase peripheral circulation of T helper 17 cells, a pro-inflammatory T cell (68,69), and a markedly decrease in CD4+CD25+ Tregs, an anti-inflammatory cell (70). TACE also altered the cytokine profiles (71), the level of T cell activation, and subpopulations of immune cells (72).
Immunomodulatory effects of tumor embolization and radiation therapy are thus opening up the possibility that such non-surgical treatment may be combined with immunotherapies.
Future perspectives
Combinatorial approaches
Hepatocellular carcinoma has a complex immunosuppressive network as discussed above. HCC is also a heterogeneous tumor with an often unpredictable and inconsistent response to treatment. Within a tumor nodule, HCC cells share a common mutation driving carcinogenesis (driver mutation) with other passenger mutations with unknown roles in carcinogenesis and progression. In addition, cells native to the liver contribute to tolerogenicity given its intrinsic anatomy and function. Sorafenib, which is immunomodulatory, is not curative, and therefore, patients treated with sorafenib have eventual disease progression. This unsatisfactory response to sorafenib monotherapy in the context of heterogeneous and immunosuppressive tumor environment suggests combinatorial approaches of immunomodulation.
As demonstrated by Kalathil et al., the combined depletion of Tregs, MDSC, and PD-1+ T cells from patients with HCC resulted in augmentation of CD8+ T-cell granzyme B production and an increase in the number of CD4+ T cells that produce IFN-γ (73). This data suggests that combination approaches may better enhance endogenous antitumor responses compared to monotherapies. With this evidence, many combinatorial approaches to treat HCC with locoregional and systemic immunosuppression have been attempted. However, one of the most important issues of combinatorial therapies is that most HCC patients have underlying liver cirrhosis. The combination of medications or methodologies could increase liver toxicity resulting in adverse outcomes. In the clinical setting it is crucial to ensure a safe liver toxicity profile when using combinatorial treatment approaches.
Liver directed therapies including local tumor ablation have been widely used for advanced HCC, and these are now known to be immunomodulatory as mentioned above. Through ablation mediated tumor destruction cellular debris can enhance tumor-specific locoregional antigen presentation (13). When combined with immune checkpoint inhibitors, the ablative therapies have the potential of having a synergistic immunomodulatory effect. Studies have aimed to quantitate the role of these liver directed therapies when combined with sorafenib or immune checkpoint inhibitors. In a pilot study, a CTLA-4 inhibitor, tremelimumab in combination with subtotal TACE or RFA in patients with advanced HCC was safe and feasible (74). One current study is investigating durvalumab (PD-1 inhibitor) and tremelimumab combined with ablation therapies including RFA, TACE, or cryoablation for patients with advanced liver or biliary tract cancer (NCT02821754). A similar study enrolls patients with HCC for treatment with tremelimumab and chemoembolization or ablation (NCT01853618). Another clinical trial aims at assessing the synergistic immunomodulatory effect of TACE and autologous immune cell therapy in HCC (NCT01828762).
Sorafenib has also been combined with tumor embolization or ablation to assess the joint immune modulating response. There are a number of clinical trials underway including NCT00919009, NCT01556815, NCT01319942, NCT00768937, NCT01605734, NCT02529761, NCT02504983, and NCT01906216. Clinical trials on the combination of sorafenib and immunotherapy are underway: sorafenib and vaccinia virus-based immunotherapy are combined (NCT02562755); and anti-PD-1 antibody, PDR001 is in combination with sorafenib (NCT02988440).
Hepatitis viral infection and HCC treatment
Patients with chronic HBV, HCV, human immunodeficiency virus (HIV), or tuberculosis have constant exposure to viral or bacterial antigens. As a consequence, the expression of PD-1, PD-L1, and PD-L2 is upregulated, and the T cell- or NK cell-mediated antiviral or antibacterial immune response is attenuated (75). In patients with chronic HBV, PD-1+ T-cells are impaired in their antiviral effector functions (76,77). Clinical studies have aimed to assess the response of HBV, HCV, and other chronic infections to immune checkpoint inhibitors, with a goal of reversing T-cell exhaustion. One such study has shown that the inhibition of PD-1 resulted in increased production of proinflammatory cytokines including IFN-γ and IL-2 in patients with chronic HBV (78). Another study showed that patients with chronic HCV had a significant decrease in the HCV viral load when they received nivolumab (79).
It is important to monitor the differential responses to immune checkpoint inhibitors for patients with HBV or HCV as well as non-infected patients with HCC, due to a risk of viral reactivation. As mentioned above, a preliminary report of CheckMate 040 (52) showed that nivolumab was well tolerated with a durable response in patients with HCC infected with HBV and HCV with no evidence of viral flare. However, recent reports have demonstrated an unexpectedly high rate of the recurrence of hepatocellular carcinoma post-treatment when hepatitis C viral treatment rapidly decreased hepatitis C viral load. It is suspected that the disruption or dysregulation of immune surveillance upon a rapid decrease in hepatitis C viral load may trigger the emergence of metastatic clones (80-83). While some studies suggest that the better control of HCV reduces the incidence of HCC, other studies suggest that the incidence of HCC increases when HCV is treated and controlled with antiviral regimens (83-85). These contradictory results seem to be associated with the complex intertwining of hepatitis virus, cirrhosis, and HCC, as well as various factors such as the degree of cirrhosis compensation, portal hypertension, and the immunosuppressive tumor microenvironment (83,84,86). Without further investigation, no clear immune system relation can be elucidated between hepatitis viral infection and HCC. Despite local and systemic complexity of the immune system in HCC with hepatitis viral infection, there is limited data about the interaction of hepatitis virus with immune checkpoint inhibitors. Therefore, when hepatitis virus-infected patients with HCC are treated with immunomodulators, it is mandatory to closely monitor the safety profile and hepatitis viral loads. It is expected that there will be more research and clinical studies on the interplay of hepatocellular carcinoma and hepatitis viral infection.
Chimeric antigen receptor T cell
The chimeric antigen receptor (CAR)-T cell is an engineered tumor-targeted T cell created through the genetic transfer of tumor antigen specific receptors. The three main elements of CAR-T cells are an extracellular antigen binding domain, a transmembrane domain, and a cytoplasmic signaling domain (87). Third generation CAR-T cells utilize at least two cytoplasmic signaling domains to promote the proliferation of CAR-T cells and anti-tumor activity (88-90). In hematologic malignancies, particularly acute lymphocytic leukemia, CAR-T cell therapy has been widely used and has shown promising results.
CAR-T cells have also been used for patients with solid cancers including ovarian cancer (91), renal cell carcinoma (92), and breast cancer (93) although there have been on-going tolerance and safety issues. Early stage clinical trials are underway for patients with HCC, but clinical data on the response rate or safety profiles have not been reported. Several clinical trials with CAR-T cells targeting different antigens are underway: mucin-1 (NCT02587689), epithelial cell adhesion molecule (EpCAM) (NCT02729493), CD133 (NCT02541370), and glypican-3 (NCT02395250, NCT02723942, and NCT02715362).
Cytokine related therapy
As discussed above, one of the main components of the immunosuppressive network in HCC is the disarray of cytokines. Several therapies have aimed to modify the cytokine environment for the treatment of HCC. In addition, there have been multiple clinical trials utilizing cytokine-induced killer cells (CIKs) (94,95). CIKs are autologous peripheral mononuclear cells created ex vivo by incubation with cytokines including IFN-γ, IL-1, IL-2, and anti-CD3 antibody. These cells bypass immune priming and T-cell activation and are able to directly target malignant cells. Ma et al. analyzed previously published data on CIKs therapy and demonstrated that CIKs therapy improved overall survival, progression free survival, overall response rate, and quality of life (95). However, a phase III study with 200 patients randomized to adjuvant CIKs or placebo demonstrated a prolonged time-to-recurrence without improvement of disease free survival or overall survival (96). There are ongoing clinical trials to assess CIKs therapy (NCT02851784, and other completed trials). Secondary to its immunomodulatory design, clinical trials have used combinatorial approaches with CIKs and anti-PD-1 therapy (NCT02886897), as well as CKIs and MWA (NCT02851784). Clinical trials with other systemic immunomodulators and CIKs are expected.
TGF-β is one of the main cytokines associated with fibrogenesis and angiogenesis. However, evidence suggests that its role is primarily as an immunosuppressive cytokine by modulating Treg cells (97). Its role in enhancing Treg activity suggests that TGF-β is one of the main components of the immunosuppressive network in HCC. One study presented at the ASCO annual meeting in 2014 provided promising evidence about TGF-β1 inhibitor in patients with advanced HCC (98). There are ongoing clinical trials, including galunisertib, a TGF-β1 inhibitor in combination with sorafenib or ramucirumab (NCT02240433, NCT01246986, and NCT02178358), nivolumab (NCT02423343), or stereotactic body radiotherapy (NCT02906397).
Oncolytic virus-based therapy
Oncolytic viruses are therapeutic viruses engineered to have cancer specific targets and replicate in cancer cells. Transthyretin-promoter-driven adenovirus (99), AFP-promoter-driven adenovirus (100), membrane metalloproteinase-activated measles virus fusion protein (101), or miR-122-regulated adenovirus (102), as well as JX-594, dl1520, H101 and VSV-hIFN-β have been used in HCC (103). In particular, JX-594 (pexastimogene devacirepvec), a modified Copenhagen strain vaccinia poxvirus has been extensively studied and showed a promising response in patients with HCC (104). Since the first clinical trial (NCT00554372), multiple trials have been completed with anticipated results (NCT01171651, NCT01636284, NCT01387555, and NCT00629759). Oncolytic viruses not only directly destroy cancer cells, but they also elicit an anti-cancer immune response acting as an immunomodulatory agent (103,105). Currently, a clinical trial (NCT02562755) is recruiting patients to compare JX-594-based immunotherapy plus sorafenib compared to sorafenib alone. Subsequent clinical trials combining oncolytic-virus-based immunotherapy with other immunomodulators are anticipated.
Biomarkers and mutational profiles of HCC
The extent to which a cancer with a certain mutational profile or PD-L1 expression responds to immunotherapy is debatable. Many ongoing research projects are utilizing genomic sequencing to better quantify responses to immunomodulators. Studies have shown that stage IV squamous cell lung cancer has a better response to nivolumab than docetaxel regardless of PD-L1 expression levels (106). However, in patients with lung adenocarcinoma and negative PD-L1 levels, survival was similar between docetaxel and nivolumab (107). JAK1/2 mutations have been shown to prevent reactive PD-L1 expression, and patients harboring such tumors would be unlikely to respond to PD-1 blockade therapy (108). In colorectal cancer, patients with mismatch repair mutations have an enhanced response to PD-1 blockade. More specifically, those with high somatic mutation loads are associated with prolonged progression-free survival with pembrolizumab (109). However, there is no known data about the response rate to PD-1 inhibitors with respect to the mutational profile or PD-L1 level in HCC. HCC is a heterogeneous tumor, and the expression of PD-L1 is multifactorial, depending on the stage of tumor, prior treatment regimens, and tumor burden. As discussed above, the microenvironment of HCC involves a complex network of immune cells and liver native cells, as well as multiple pro- or anti-inflammatory cytokines. Therefore, the PD-L1 expression level on HCC tumor needs to be assessed along with these factors for clinical trial enrollment (13,110).
Conclusions
Hepatocellular carcinoma is a chemorefractory malignancy and challenging to treat. The treatment of choice for early stage HCC is surgery or locoregional therapies. Sorafenib is the only FDA-approved medication for advanced HCC, with proven immunomodulatory effects. Recent studies have demonstrated that HCC has a complex immunosuppressive network and tumor microenvironment. Therefore, immunomodulation in HCC has become a main focus of treatment. Early phase trials with monotherapy of an immune checkpoint inhibitor or in combination with other treatment modalities have shown promise across etiologies, and multiple clinical trials are now in progress. In secondary prevention and adjuvant setting, only antiviral therapy has been successful; the role of immunomodulation needs further investigation. Combinatorial approaches and optimal sequencing to target the heterogeneous, immunosuppressive tumor are expected to be the main avenue of future HCC treatment in the future.
Acknowledgements
None.
Footnote
Conflicts of Interest: The authors have no conflicts of interest to declare.
References
- Skolnick AA. Armed with epidemiologic research, China launches programs to prevent liver cancer. JAMA 1996;276:1458-9. [Crossref] [PubMed]
- Davila JA, Morgan RO, Shaib Y, et al. Hepatitis C infection and the increasing incidence of hepatocellular carcinoma: a population-based study. Gastroenterology 2004;127:1372-80. [Crossref] [PubMed]
- El-Serag HB, Kanwal F. Epidemiology of hepatocellular carcinoma in the United States: where are we? Where do we go? Hepatology 2014;60:1767-75. [Crossref] [PubMed]
- El-Serag HB. Hepatocellular carcinoma. N Engl J Med 2011;365:1118-27. [Crossref] [PubMed]
- Nagahama H, Okada S, Okusaka T, et al. Predictive factors for tumor response to systemic chemotherapy in patients with hepatocellular carcinoma. Jpn J Clin Oncol 1997;27:321-4. [Crossref] [PubMed]
- Torres HA, Davila M. Reactivation of hepatitis B virus and hepatitis C virus in patients with cancer. Nat Rev Clin Oncol 2012;9:156-66. [Crossref] [PubMed]
- Nowak AK, Chow PK, Findlay M. Systemic therapy for advanced hepatocellular carcinoma: a review. Systemic therapy for advanced hepatocellular carcinoma: a review. Eur J Cancer 2004;40:1474-84. [Crossref] [PubMed]
- Abou-Alfa GK, Schwartz L, Ricci S, et al. Phase II study of sorafenib in patients with advanced hepatocellular carcinoma. J Clin Oncol 2006;24:4293-300. [Crossref] [PubMed]
- Prieto J, Melero I, Sangro B. Immunological landscape and immunotherapy of hepatocellular carcinoma. Nat Rev Gastroenterol Hepatol 2015;12:681-700. [Crossref] [PubMed]
- Flecken T, Schmidt N, Hild S, et al. Immunodominance and functional alterations of tumor-associated antigen-specific CD8+ T-cell responses in hepatocellular carcinoma. Hepatology 2014;59:1415-26. [Crossref] [PubMed]
- Pardoll DM. The blockade of immune checkpoints in cancer immunotherapy. Nat Rev Cancer 2012;12:252-64. [Crossref] [PubMed]
- Jenne CN, Kubes P. Immune surveillance by the liver. Nat Immunol 2013;14:996-1006. [Crossref] [PubMed]
- Harding JJ, El Dika I, Abou-Alfa GK. Immunotherapy in hepatocellular carcinoma: Primed to make a difference? Cancer 2016;122:367-77. [Crossref] [PubMed]
- Tagliamonte M, Petrizzo A, Tornesello ML, et al. Combinatorial immunotherapy strategies for hepatocellular carcinoma. Curr Opin Immunol 2016;39:103-13. [Crossref] [PubMed]
- Buonaguro L, Petrizzo A, Tagliamonte M, et al. Challenges in cancer vaccine development for hepatocellular carcinoma. J Hepatol 2013;59:897-903. [Crossref] [PubMed]
- Thomson AW, Knolle PA. Antigen-presenting cell function in the tolerogenic liver environment. Nat Rev Immunol 2010;10:753-66. [Crossref] [PubMed]
- Schurich A, Khanna P, Lopes AR, et al. Role of the coinhibitory receptor cytotoxic T lymphocyte antigen-4 on apoptosis-Prone CD8 T cells in persistent hepatitis B virus infection. Hepatology 2011;53:1494-503. [Crossref] [PubMed]
- Nakamoto N, Kaplan DE, Coleclough J, et al. Functional restoration of HCV-specific CD8 T cells by PD-1 blockade is defined by PD-1 expression and compartmentalization. Gastroenterology 2008;134:1927-37. [Crossref] [PubMed]
- Schildberg FA, Hegenbarth SI, Schumak B, et al. Liver sinusoidal endothelial cells veto CD8 T cell activation by antigen-presenting dendritic cells. Eur J Immunol 2008;38:957-67. [Crossref] [PubMed]
- Knolle PA, Germann T, Treichel U, et al. Endotoxin down-regulates T cell activation by antigen-presenting liver sinusoidal endothelial cells. J Immunol 1999;162:1401-7. [PubMed]
- Heymann F, Peusquens J, Ludwig-Portugall I, et al. Liver inflammation abrogates immunological tolerance induced by Kupffer cells. Hepatology 2015;62:279-91. [Crossref] [PubMed]
- Pillarisetty VG, Shah AB, Miller G, et al. Liver dendritic cells are less immunogenic than spleen dendritic cells because of differences in subtype composition. J Immunol 2004;172:1009-17. [Crossref] [PubMed]
- De Luca A, Gallo M, Aldinucci D, et al. Role of the EGFR ligand/receptor system in the secretion of angiogenic factors in mesenchymal stem cells. J Cell Physiol 2011;226:2131-8. [Crossref] [PubMed]
- Hato T, Goyal L, Greten TF, et al. Immune checkpoint blockade in hepatocellular carcinoma: current progress and future directions. Hepatology 2014;60:1776-82. [Crossref] [PubMed]
- Zhang Z, Zhang Y, Sun XX, et al. microRNA-146a inhibits cancer metastasis by downregulating VEGF through dual pathways in hepatocellular carcinoma. Mol Cancer 2015;14:5. [Crossref] [PubMed]
- Colegio OR, Chu NQ, Szabo AL, et al. Functional polarization of tumour-associated macrophages by tumour-derived lactic acid. Nature 2014;513:559-63. [Crossref] [PubMed]
- Budhu A, Forgues M, Ye QH, et al. Prediction of venous metastases, recurrence, and prognosis in hepatocellular carcinoma based on a unique immune response signature of the liver microenvironment. Cancer Cell 2006;10:99-111. [Crossref] [PubMed]
- Li M, Sun R, Xu L, et al. Kupffer Cells Support Hepatitis B Virus-Mediated CD8+ T Cell Exhaustion via Hepatitis B Core Antigen-TLR2 Interactions in Mice. J Immunol 2015;195:3100-9. [Crossref] [PubMed]
- Tinoco R, Alcalde V, Yang Y, et al. Cell-intrinsic transforming growth factor-beta signaling mediates virus-specific CD8+ T cell deletion and viral persistence in vivo. Immunity 2009;31:145-57. [Crossref] [PubMed]
- Llovet JM, Ricci S, Mazzaferro V, et al. Sorafenib in advanced hepatocellular carcinoma. N Engl J Med 2008;359:378-90. [Crossref] [PubMed]
- Raoul JL, Bruix J, Greten TF, et al. Relationship between baseline hepatic status and outcome, and effect of sorafenib on liver function: SHARP trial subanalyses. J Hepatol 2012;56:1080-8. [Crossref] [PubMed]
- Houben R, Voigt H, Noelke C, et al. MAPK-independent impairment of T-cell responses by the multikinase inhibitor sorafenib. Mol Cancer Ther 2009;8:433-40. [Crossref] [PubMed]
- Heine A, Held SA, Bringmann A, et al. Immunomodulatory effects of anti-angiogenic drugs. Leukemia 2011;25:899-905. [Crossref] [PubMed]
- Chuang HY, Chang YF, Liu RS, et al. Serial Low Doses of Sorafenib Enhance Therapeutic Efficacy of Adoptive T Cell Therapy in a Murine Model by Improving Tumor Microenvironment. PLoS One 2014;9:e109992. [Crossref] [PubMed]
- Cao M, Xu Y, Youn JI, et al. Kinase inhibitor Sorafenib modulates immunosuppressive cell populations in a murine liver cancer model. Lab Invest 2011;91:598-608. [Crossref] [PubMed]
- Chen ML, Yan BS, Lu WC, et al. Sorafenib relieves cell-intrinsic and cell-extrinsic inhibitions of effector T cells in tumor microenvironment to augment antitumor immunity. Int J Cancer 2014;134:319-31. [Crossref] [PubMed]
- Alfaro C, Suarez N, Gonzalez A, et al. Influence of bevacizumab, sunitinib and sorafenib as single agents or in combination on the inhibitory effects of VEGF on human dendritic cell differentiation from monocytes. Br J Cancer 2009;100:1111-9. [Crossref] [PubMed]
- Sprinzl MF, Reisinger F, Puschnik A, et al. Sorafenib perpetuates cellular anticancer effector functions by modulating the crosstalk between macrophages and natural killer cells. Hepatology 2013;57:2358-68. [Crossref] [PubMed]
- Cabrera R, Ararat M, Xu Y, et al. Immune modulation of effector CD4+ and regulatory T cell function by sorafenib in patients with hepatocellular carcinoma. Cancer Immunol Immunother 2013;62:737-46. [Crossref] [PubMed]
- Kalathil SG, Lugade AA, Miller A, et al. PD-1+ and Foxp3+ T cell reduction correlates with survival of HCC patients after sorafenib therapy. JCI Insight 2016;1:e86182. [Crossref] [PubMed]
- Urosevic-Maiwald M, Barysch MJ, Cheng PF, et al. In vivo profiling reveals immunomodulatory effects of sorafenib and dacarbazine on melanoma. Oncoimmunology 2015;4:e988458. [Crossref] [PubMed]
- Freeman GJ, Long AJ, Iwai Y, et al. Engagement of the PD-1 immunoinhibitory receptor by a novel B7 family member leads to negative regulation of lymphocyte activation. J Exp Med 2000;192:1027-34. [Crossref] [PubMed]
- Okazaki T, Honjo T. PD-1 and PD-1 ligands: from discovery to clinical application. Int Immunol 2007;19:813-24. [Crossref] [PubMed]
- Dunn GP, Bruce AT, Ikeda H, et al. Cancer immunoediting: from immunosurveillance to tumor escape. Nat Immunol 2002;3:991-8. [Crossref] [PubMed]
- Spranger S, Spaapen RM, Zha Y, et al. Up-regulation of PD-L1, IDO, and T(regs) in the melanoma tumor microenvironment is driven by CD8(+) T cells. Sci Transl Med 2013;5:200ra116. [Crossref] [PubMed]
- Kinter AL, Godbout EJ, McNally JP, et al. The common gamma-chain cytokines IL-2, IL-7, IL-15, and IL-21 induce the expression of programmed death-1 and its ligands. J Immunol 2008;181:6738-46. [Crossref] [PubMed]
- Pardoll DM. Immunology beats cancer: a blueprint for successful translation. Nat Immunol 2012;13:1129-32. [Crossref] [PubMed]
- Topalian SL, Hodi FS, Brahmer JR, et al. Safety, activity, and immune correlates of anti-PD-1 antibody in cancer. N Engl J Med 2012;366:2443-54. [Crossref] [PubMed]
- Horvat TZ, Adel NG, Dang TO, et al. Immune-Related Adverse Events, Need for Systemic Immunosuppression, and Effects on Survival and Time to Treatment Failure in Patients With Melanoma Treated With Ipilimumab at Memorial Sloan Kettering Cancer Center. J Clin Oncol 2015;33:3193-8. [Crossref] [PubMed]
- Weber JS, Hodi FS, Wolchok JD, et al. Safety Profile of Nivolumab Monotherapy: A Pooled Analysis of Patients With Advanced Melanoma. J Clin Oncol 2017;35:785-92. [Crossref] [PubMed]
- Sangro B, Gomez-Martin C, de la Mata M, et al. A clinical trial of CTLA-4 blockade with tremelimumab in patients with hepatocellular carcinoma and chronic hepatitis C. J Hepatol 2013;59:81-8. [Crossref] [PubMed]
- El-Khoueiry AB, Melero I, Crocenzi TS, et al. Phase I/II safety and antitumor activity of nivolumab in patients with advanced hepatocellular carcinoma (HCC): CA209-040. J Clin Oncol 2015;33:abstr LBA101.
- Javle M. Hepatobiliary Cancer; HCC: Checkpoint Inhibition. Discussant of Abstract 226; Proc Amer Soc Clin Oncol Gastrointest Canc Sym 2017.
- Sangro B, Park JW, Cruz CM, et al. A randomized, multicenter, phase 3 study of nivolumab vs sorafenib as first-line treatment in patients (pts) with advanced hepatocellular carcinoma (HCC): CheckMate-459. J Clin Oncol 2016;34:abstr TPS4147.
- Kudo M. Immune Checkpoint Blockade in Hepatocellular Carcinoma: 2017 Update. Liver Cancer 2016;6:1-12. [Crossref] [PubMed]
- Schueller G, Kettenbach J, Sedivy R, et al. Heat shock protein expression induced by percutaneous radiofrequency ablation of hepatocellular carcinoma in vivo. Int J Oncol 2004;24:609-13. [PubMed]
- Ali MY, Grimm CF, Ritter M, et al. Activation of dendritic cells by local ablation of hepatocellular carcinoma. J Hepatol 2005;43:817-22. [Crossref] [PubMed]
- Ahmad F, Gravante G, Bhardwaj N, et al. Changes in interleukin-1β and 6 after hepatic microwave tissue ablation compared with radiofrequency, cryotherapy and surgical resections. Am J Surg 2010;200:500-6. [Crossref] [PubMed]
- Teng LS, Jin KT, Han N, et al. Radiofrequency ablation, heat shock protein 70 and potential anti-tumor immunity in hepatic and pancreatic cancers: a minireview. Hepatobiliary Pancreat Dis Int 2010;9:361-5. [PubMed]
- Haen SP, Gouttefangeas C, Schmidt D, et al. Elevated serum levels of heat shock protein 70 can be detected after radiofrequency ablation. Cell Stress Chaperones 2011;16:495-504. [Crossref] [PubMed]
- Ito F, Ku AW, Bucsek MJ, et al. Immune Adjuvant Activity of Pre-Resectional Radiofrequency Ablation Protects against Local and Systemic Recurrence in Aggressive Murine Colorectal Cancer. PLoS One 2015;10:e0143370. [Crossref] [PubMed]
- Jansen MC, van Hillegersberg R, Chamuleau RA, et al. Outcome of regional and local ablative therapies for hepatocellular carcinoma: a collective review. Eur J Surg Oncol 2005;31:331-47. [Crossref] [PubMed]
- Jansen MC, van Hillegersberg R, Schoots IG, et al. Cryoablation induces greater inflammatory and coagulative responses than radiofrequency ablation or laser induced thermotherapy in a rat liver model. Surgery 2010;147:686-95. [Crossref] [PubMed]
- Sabel MS, Nehs MA, Su G, et al. Immunologic response to cryoablation of breast cancer. Breast Cancer Res Treat 2005;90:97-104. [Crossref] [PubMed]
- Gravante G, Sconocchia G, Ong SL, et al. Immunoregulatory effects of liver ablation therapies for the treatment of primary and metastatic liver malignancies. Liver Int 2009;29:18-24. [Crossref] [PubMed]
- Lencioni R, Chen XP, Dagher L, et al. Treatment of intermediate/advanced hepatocellular carcinoma in the clinic: how can outcomes be improved? Oncologist 2010;15 Suppl 4:42-52. [Crossref] [PubMed]
- Llovet JM, Real MI, Montaña X, et al. Arterial embolisation or chemoembolisation versus symptomatic treatment in patients with unresectable hepatocellular carcinoma: a randomised controlled trial. Lancet 2002;359:1734-9. [Crossref] [PubMed]
- Liao Y, Wang B, Huang ZY, et al. Increased Circulating Th17 Cells after Transarterial Chemoembolization Correlate with Improved Survival in Stage III Hepatocellular Carcinoma: A Prospective Study. PLoS One 2013;8:e60444. [Crossref] [PubMed]
- Ranieri G, Marech I, Lorusso V, et al. Molecular targeting agents associated with transarterial chemoembolization or radiofrequency ablation in hepatocarcinoma treatment. World J Gastroenterol 2014;20:486-97. [Crossref] [PubMed]
- Liao J, Xiao J, Zhou Y, et al. Effect of transcatheter arterial chemoembolization on cellular immune function and regulatory T cells in patients with hepatocellular carcinoma. Mol Med Rep 2015;12:6065-71. [Crossref] [PubMed]
- Ikei S, Ogawa M, Beppu T, et al. Changes in IL-6, IL-8, C-reactive protein and pancreatic secretory trypsin inhibitor after transcatheter arterial chemo-embolization therapy for hepato-cellular carcinoma. Cytokine 1992;4:581-4. [Crossref] [PubMed]
- Ayaru L, Pereira SP, Alisa A, et al. Unmasking of alpha-fetoprotein-specific CD4(+) T cell responses in hepatocellular carcinoma patients undergoing embolization. J Immunol 2007;178:1914-22. [Crossref] [PubMed]
- Kalathil S, Lugade AA, Miller A, et al. Higher Frequencies of GARP+CTLA-4+Foxp3+ T Regulatory Cells and Myeloid-Derived Suppressor Cells in Hepatocellular Carcinoma Patients Are Associated with Impaired T-Cell Functionality. Cancer Res 2013;73:2435-44. [Crossref] [PubMed]
- Duffy A, Makarova-Rusher O, Kerkar S, et al. A pilot study of tremelimumab-a monoclonal antibody against CTLA-4–in combination with either trans catheter arterial chemoembolization (TACE) or radiofrequency ablation (RFA) in patients with hepatocellular carcinoma (HCC). J Clin Oncol 2015;33:abstr 4081.
- Kasprowicz V, Schulze Zur Wiesch J, Kuntzen T, et al. High level of PD-1 expression on hepatitis C virus (HCV)-specific CD8+ and CD4+ T cells during acute HCV infection, irrespective of clinical outcome. J Virol 2008;82:3154-60. [Crossref] [PubMed]
- Lopes AR, Kellam P, Das A, et al. Bim-mediated deletion of antigen-specific CD8 T cells in patients unable to control HBV infection. J Clin Invest 2008;118:1835-45. [Crossref] [PubMed]
- Rao M, Valentini D, Dodoo E, et al. Anti-PD-1/PD-L1 therapy for infectious diseases: learning from the cancer paradigm. Int J Infect Dis 2017;56:221-8. [Crossref] [PubMed]
- Fisicaro P, Valdatta C, Massari M, et al. Antiviral intrahepatic T-cell responses can be restored by blocking programmed death-1 pathway in chronic hepatitis B. Gastroenterology 2010;138:682-93. [Crossref] [PubMed]
- Gardiner D, Lalezari J, Lawitz E, et al. A randomized, double-blind, placebo-controlled assessment of BMS-936558, a fully human monoclonal antibody to programmed death-1 (PD-1), in patients with chronic hepatitis C virus infection. PLoS One 2013;8:e63818. [Crossref] [PubMed]
- Reig M, Mariño Z, Perelló C, et al. Unexpected high rate of early tumor recurrence in patients with HCV-related HCC undergoing interferon-free therapy. J Hepatol 2016;65:719-26. [Crossref] [PubMed]
- Tsai PC, Huang CF, Yu ML. Unexpected early tumor recurrence in patients with hepatitis C virus-related hepatocellular carcinoma undergoing interferon-free therapy: Issue of the interval between HCC treatment and antiviral therapy. J Hepatol 2017;66:464. [Crossref] [PubMed]
- Nault JC, Colombo M. Hepatocellular carcinoma and direct acting antiviral treatments: Controversy after the revolution. J Hepatol 2016;65:663-5. [Crossref] [PubMed]
- D'Ambrosio R, Colombo M. Should surveillance for liver cancer be modified in hepatitis C patients after treatment-related cirrhosis regression? Liver Int 2016;36:783-90. [Crossref] [PubMed]
- van der Meer AJ, Veldt BJ, Feld JJ, et al. Association between sustained virological response and all-cause mortality among patients with chronic hepatitis C and advanced hepatic fibrosis. JAMA 2012;308:2584-93. [Crossref] [PubMed]
- Fattovich G, Stroffolini T, Zagni I, Donato F. Hepatocellular carcinoma in cirrhosis: incidence and risk factors. Gastroenterology 2004;127:S35-50. [Crossref] [PubMed]
- Foster GR, Irving WL, Cheung MC, et al. Impact of direct acting antiviral therapy in patients with chronic hepatitis C and decompensated cirrhosis. J Hepatol 2016;64:1224-31. [Crossref] [PubMed]
- Jin C, Fotaki G, Ramachandran M, et al. Safe engineering of CAR T cells for adoptive cell therapy of cancer using long-term episomal gene transfer. EMBO Mol Med 2016;8:702-11. [Crossref] [PubMed]
- Hong YP, Li ZD, Prasoon P, Zhang Q. Immunotherapy for hepatocellular carcinoma: From basic research to clinical use. World J Hepatol 2015;7:980-92. [Crossref] [PubMed]
- Grupp SA, Kalos M, Barrett D, et al. Chimeric antigen receptor-modified T cells for acute lymphoid leukemia. N Engl J Med 2013;368:1509-18. [Crossref] [PubMed]
- Porter DL, Levine BL, Kalos M, et al. Chimeric antigen receptor-modified T cells in chronic lymphoid leukemia. N Engl J Med 2011;365:725-33. [Crossref] [PubMed]
- Kershaw MH, Westwood JA, Parker LL, et al. A phase I study on adoptive immunotherapy using gene-modified T cells for ovarian cancer. Clin Cancer Res 2006;12:6106-15. [Crossref] [PubMed]
- Lamers CH, Klaver Y, Gratama JW, et al. Treatment of metastatic renal cell carcinoma (mRCC) with CAIX CAR-engineered T-cells-a completed study overview. Biochem Soc Trans 2016;44:951-9. [Crossref] [PubMed]
- Song DG, Ye Q, Poussin M, et al. Effective adoptive immunotherapy of triple-negative breast cancer by folate receptor-alpha redirected CAR T cells is influenced by surface antigen expression level. J Hematol Oncol 2016;9:56. [Crossref] [PubMed]
- Lee JH, Lee JH, Lim YS, et al. Adjuvant immunotherapy with autologous cytokine-induced killer cells for hepatocellular carcinoma. Gastroenterology 2015;148:1383-91.e6. [Crossref] [PubMed]
- Ma Y, Xu YC, Tang L, et al. Cytokine-induced killer (CIK) cell therapy for patients with hepatocellular carcinoma: efficacy and safety. Exp Hematol Oncol 2012;1:11. [Crossref] [PubMed]
- Xu L, Wang J, Kim Y, et al. A randomized controlled trial on patients with or without adjuvant autologous cytokine-induced killer cells after curative resection for hepatocellular carcinoma. Oncoimmunology 2015;5:e1083671. [Crossref] [PubMed]
- Shen Y, Wei Y, Wang Z, et al. TGF-β regulates hepatocellular carcinoma progression by inducing Treg cell polarization. Cell Physiol Biochem 2015;35:1623-32. [Crossref] [PubMed]
- Faivre SJ, Santoro A, Kelley RK et al. A phase 2 study of a novel transforming growth factor-beta (TGF-β1) receptor I kinase inhibitor, LY2157299 monohydrate (LY), in patients with advanced hepatocellular carcinoma (HCC). J Clin Oncol 2014;32:abstr LBA173.
- Hsieh JL, Lee CH, Teo ML, et al. Transthyretin-driven oncolytic adenovirus suppresses tumor growth in orthotopic and ascites models of hepatocellular carcinoma. Cancer Sci 2009;100:537-45. [Crossref] [PubMed]
- Zhang KJ, Qian J, Wang SB, Yang Y. Targeting Gene-Viro-Therapy with AFP driving Apoptin gene shows potent antitumor effect in hepatocarcinoma. J Biomed Sci 2012;19:20. [Crossref] [PubMed]
- Mühlebach MD, Schaser T, Zimmermann M, et al. Liver cancer protease activity profiles support therapeutic options with matrix metalloproteinase-activatable oncolytic measles virus. Cancer Res 2010;70:7620-9. [Crossref] [PubMed]
- Cawood R, Chen HH, Carroll F, et al. Use of tissue-specific microRNA to control pathology of wild-type adenovirus without attenuation of its ability to kill cancer cells. PLoS Pathog 2009;5:e1000440. [Crossref] [PubMed]
- Jebar AH, Errington-Mais F, Vile RG, et al. Progress in clinical oncolytic virus-based therapy for hepatocellular carcinoma. J Gen Virol 2015;96:1533-50. [Crossref] [PubMed]
- Heo J, Reid T, Ruo L, et al. Randomized dose-finding clinical trial of oncolytic immunotherapeutic vaccinia JX-594 in liver cancer. Nat Med 2013;19:329-36. [Crossref] [PubMed]
- Melcher A, Parato K, Rooney CM, Bell JC. Thunder and lightning: immunotherapy and oncolytic viruses collide. Mol Ther 2011;19:1008-16. [Crossref] [PubMed]
- Brahmer J, Reckamp KL, Baas P, et al. Nivolumab versus docetaxel in advanced squamous-cell non-small-cell lung cancer. N Engl J Med 2015;373:123-35. [Crossref] [PubMed]
- Borghaei H, Paz-Ares L, Horn L, et al. Nivolumab versus Docetaxel in Advanced Nonsquamous Non-Small-Cell Lung Cancer. N Engl J Med 2015;373:1627-39. [Crossref] [PubMed]
- Shin DS, Zaretsky JM, Escuin-Ordinas H, et al. Primary Resistance to PD-1 Blockade Mediated by JAK1/2 Mutations. Cancer Discov 2017;7:188-201. [Crossref] [PubMed]
- Le DT, Uram JN, Wang H, et al. PD-1 Blockade in Tumors with Mismatch-Repair Deficiency. N Engl J Med 2015;372:2509-20. [Crossref] [PubMed]
- Noman MZ, Desantis G, Janji B, et al. PD-L1 is a novel direct target of HIF-1α, and its blockade under hypoxia enhanced MDSC-mediated T cell activation. J Exp Med 2014;211:781-90. [Crossref] [PubMed]