Systemic therapy for advanced hepatocellular carcinoma: an update
Introduction
Hepatocellular carcinoma (HCC) is the second and sixth leading cause of cancer related mortality in men and women, respectively (1). The annual incidence is similar to the yearly death rate. Despite advances in our understanding of the molecular pathways involved in HCC, our therapeutic armamentarium remains limited and survival dismal. In early stage disease [Barcelona clinic liver cancer (BCLC) stages 0 and A], surgical resection, ablation methods, and liver transplantation reportedly extend survival by more than 5 years. However, less than half of patients have resectable tumors at initial presentation and, even after successful resection, 70% will eventually relapse due to micrometastases and de novo malignant transformation of adjacent liver cells (2,3). Although liver transplantation remains the favored surgical modality, owing to lower risk of recurrence and improved long-term survival due to removal of the cirrhotic liver, its use is not without limitations. Risks associated with hepatic decompensation and neoplastic progression while awaiting transplant, as well as lifelong immunosuppression post-transplant increase morbidity and mortality. Thus, curative surgery is a limited option for patients with HCC. Furthermore, there are currently no effective adjuvant therapies. To date, there are only two approved therapies for intermediate and advanced stage HCC: transcatheter arterial chemoembolization (TACE) and the multi-kinase inhibitor, sorafenib. TACE extends survival to approximately 2 years in patients with intermediate stage disease, although high rates of treatment failure and risk of precipitating liver failure exist in patients with advanced cirrhosis (4). Sorafenib extends survival of patients with advanced stage disease, as shown in the landmark trials SHARP [OS: 10.7 vs. 7.9; hazard ratio (HR), 0.69; 95% confidence interval (CI), 0.55–0.87; P<0.001] and Asia-Pacific (OS: 6.5 vs. 4.2; HR, 0.68; 95% CI, 0.50–0.93; P=0.014), with a favorable safety profile (5,6). This modest but significant improvement in mortality ushered in a new era for advanced HCC, where clinical trial efforts are largely focused on targeted therapies involving multiple pathways, most commonly related to tyrosine-kinase growth factor receptors. Clinical trials have compared a variety of novel agents to sorafenib—in the first-line treatment of advanced HCC—or placebo—as salvage therapy following progression from Sorafenib treatment, sometimes in combination with TACE. However, outcomes have been disappointing, resulting in either inferior or comparable survival with a greater number of adverse effects. Of the many compounds tested for the treatment of patients with HCC, only a handful may have significant impact on survival. In this review we present some of the recent advances in HCC genomics and molecular pathway discovery and exploitation, as well as recently completed and ongoing clinical trials. We discuss the challenges and current trends in the pursuit of more effective treatments of advanced HCC.
Genetic alterations and molecular targets in HCC
HCC is a biologically complex and heterogeneous disease. Most commonly it arises from hepatitis C virus (HCV), hepatitis B virus (HBV), alcohol abuse, and other causes of liver cirrhosis. In the process of liver cirrhosis, it is believed that chronic inflammation, continuous cycles of damage and regeneration, and the presence of etiology-specific carcinogens produce cumulative genetic derangements that are involved in the hepatocarcinogenic process. There are, on average, 30 to 40 mutations per liver tumor, and 5 to 8 of these are thought to be driver mutations (7). Several studies used deep genome sequencing to identify mutations in key genes thought to be involved in various pathways critical to normal cellular homeostasis, including telomerase reverse transcriptase (TERT) expression, chromatin remodeling, oxidative stress, cell cycle/TP53, Wnt/β-catenin, hepatic differentiation, growth factor/angiogenic receptor (EGF, IGF, c-MET, PDGF, FGF, VEGF), RAS/RAF/MAPK, PI3K/AKT/mTOR, and IL6/JAK/STAT (8-11). The most common gene mutations found in HCC are TERT promoter, CTNNB1, TP53, and ARID1A (Table 1). Studies have also identified alterations in pathways that are involved in the interaction between tumor cells and their microenvironment, such as overexpression of programmed cell death-ligand-1 (PD-L1), which binds to lymphocyte programmed cell death protein-1 (PD-1) and induces immune tolerance (12). Transcriptional profiling of a large number of patient samples revealed the relative prevalence of genetic alterations in HCC; some of these mutations can potentially serve as biomarkers to direct drug development and clinical trials (Table 1) (13).
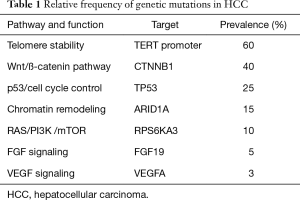
Full table
Genetic mutations in HCC often result in the overexpression and/or continuous activation of a multitude of signaling pathways. There are two genomic subclasses of HCC phenotype based on shared molecular features: proliferative and non-proliferative (14).
The proliferative subclass is more aggressive and has a higher alpha feta protein (AFP). Histologically, poorly differentiated cells are associated with the proliferative subclass, which has a greater number of gene signatures that are associated with a poor prognosis. The pathways involved in the proliferative HCC subclass include mTOR, MET, RAS, IGF, and Notch. This subclass can then be further divided into two categories: one is led by activation of the Wnt/TGF-β pathway, and the other involves the keratin, type I cytoskeletal 19 protein (a 40 kDa protein that in humans is encoded by the KRT19 gene) and an epithelial cell adhesion molecule (EpCAM), both progenitor-cell group markers.
The non-proliferative subclass has gene signatures that are associated with more normal liver function and better prognosis than the proliferative subclass. The non-proliferative subclass is correlated with better outcomes, lower AFP levels, and good-to-moderate differentiation.
The proliferative subclass usually consists of HCC secondary to HBV, while the non-proliferative subclass consists of HCC secondary to HCV infection and alcoholic cirrhosis (7).
Wnt/β-catenin pathway
The Wnt pathway is one of the primary pathways affected in the proliferative subgroup of HCC described above. In normal cellular homeostasis, in the absence of Wnt receptor ligands, cytosolic β-catenin is continuously phosphorylated and degraded in the cellular cytosol. This degradation is completed by a multiprotein complex, which includes the tumor suppressor proteins, APC and Axin, and results in proteolysis (15). Conversely, when the Wnt receptor forms a complex with the transmembrane domain Frizzled protein and a LDL receptor-related protein family (LRP5 or LRP6), the β-catenin destruction complex is inactivated and consequently β-catenin is not phosphorylated. The β-catenin then accumulates in the cytoplasm and nucleus and, in the nucleus, binds to TCF/LEF, a DNA binding protein, which then causes regulation of transcription of numerous target genes. In addition, the presence of non-phosphorylated β-catenin in the cytosol decreases cell-to-cell adhesion, which, for the most part, depends on the integrity of the e-cadherin-β-catenin-α-catenin complex formed in this process.
CTNNB1 is a common mutation in HCC, which results in continuous activation of the WNT pathway. This, in turn, results in decreased cellular adhesion which then leads to tumor proliferation, metastasis, and consequently progression of HCC (7,16). The Wnt/β-catenin pathway can be activated not only by mutations in the pathway components, but also by soluble Wnt signaling antagonists that cause excessive signaling and activation of the pathway. Examples of these antagonists include sFRP, Dkk, and Wnt-inhibitory factor (WIF-1). However, high levels of β-catenin can be seen in normal healthy liver parenchyma and are correlated with metabolic cell fate more than hepatocyte proliferation. Abnormally high levels of Wnt/β-catenin signaling are still able to cause hepatocyte proliferation, leading to liver tumor progression. Also, if hepatocyte proliferation is inhibited, Wnt/β-catenin allows for recruitment of stem-like precursor cells for growth of hepatic parenchyma. It is clear that the Wnt/β-catenin pathway is involved in hepatic tumorigenesis but the degree of involvement is unclear. β-catenin mutations have been reported to occur in 8% to 44% of HCC (median, 20%). The Wnt/β-catenin pathway is associated more with HCV-related HCC than HBV-related HCC and could be a good target for future therapeutic strategies, although there are no therapies at this time (15).
PI3K pathway
The phosphoinositide 3 kinase (PI3K) pathway also plays an important role in the proliferative subgroup of HCC by allowing for cell survival and proliferation (7,15). PI3K is an intracellular transducer enzyme with a regulatory p85 subunit that can interact with phosphotyrosines and activate enzymatic activities (17,18). AKT is one of the downstream kinases of PI3K and, upon activation, is responsible for the phosphorylation and regulation of many downstream substrates. When a downstream target of PI3K pathway, mTOR (the mechanistic target of rapamycin) is activated by AKT, it leads to protein synthesis, lipogenesis, and energy metabolism. This pathway is active in 40–50% of HCCs and is also seen in breast, colon, bile duct and lung carcinomas. It is correlated with poorer prognosis, less tumor differentiation, and earlier recurrence of cancer (15,17,18).
c-MET pathway
c-MET is a proto-oncogene that contributes to the progression of HCC. It codes for the hepatocyte growth factor receptor, which, on binding to the hepatocyte growth factor (HGF), leads to downstream activation of the MAPK and PI3K pathways. This then promotes hepatocyte proliferation and regeneration leading to progression of HCC. HGF is responsible for liver protection and is increased when there is liver tissue damage and injury. It also plays an anti-apoptotic role in liver cancer progression. HGF is likely recruited from bone marrow-derived liver sinusoidal endothelial cell progenitor cells. The c-MET receptor can also be activated by gene amplification, overexpression, or activating mutations. Besides the induction of the PI3K/AKT pathways, c-MET plays a major role in the induction of the RAS/RAF pathway, which upon translocation to the nucleus regulates a large number of genes that are related to proliferation, cell motility, and cell cycle progression. When c-MET activation results in stimulation of the rat sarcoma viral oncogene homolog, this binds with Grb2 and Son of Sevenless (SOS) and can result in RAS and downstream RAF kinase activation, followed by MEK (an effector kinase) activation, which eventually results in MAPK phosphorylation and activation. MAPK is then able to undergo nuclear translocation and perform its gene regulatory function (19-21). The activation of c-MET signaling is tightly regulated; disruption of c-MET regulation and its effect on other signaling pathways has a major effect on tumor genesis and proliferation. c-MET activity is necessary for malignant transformation, including dimerization, autophosphorylation, and kinase activity. c-MET transcription is increased in more than 30% of HCC tumors, compared with surrounding normal liver parenchyma. However, HGF expression is decreased in HCC when compared with normal liver parenchyma (22,23). In vitro studies showed that treating c-MET-overexpressing HCC cells with the c-MET inhibitor, PHA665752, led to decreased cellular growth and proliferation. This effect was not observed in HCC cells that had low expression of c-MET, indicating that c-MET is a good target for therapeutic strategies in those tumors with high expression of c-MET. This finding is supported by data from studies with Tivantinib (ARQ197), a selective oral small molecule c-MET protein inhibitor, which is discussed later in this article (24). Other possible therapies include those that target HGF (e.g., rilotumumab, ficlatuzumab), as well as those that target the extracellular domain of cMET (e.g., MetMab) (25).
VEGF pathway
The vascular endothelial growth factor (VEGF) pathway plays an essential role in angiogenesis, as well as vasculogenesis through endothelial cell proliferation and apoptosis. In addition the VEGF signaling cascade plays a role in cellular migration (7,15,26). Progression to cirrhosis has been linked to increased growth factor expression. This includes VEGF and epidermal growth factor receptor (EGFR), as well as HGF, platelet-derived growth factor (PDGF) and insulin-like growth factor (IGF).
There are three main subtypes of VEGF receptors: VEGFR-1, VEGFR-2, and VEGFR-3 (27). Binding of ligand to one of these receptors results in dimerization and auto phosphorylation of the intracellular tyrosine sites. These phosphorylated tyrosine regions then serve as docking sites for a variety of signal transduction proteins. The role of VEGF1 signaling has not been completely established but the most current supported theory is that VEGF1 activation plays a major role in the modulation of VEGF2 (27). Research has also shown that VEGF1 positive cells, which are derived from the bone marrow, might play a substantial role in different types of tumor progression (28). VEGF2 is the most studied and best understood VEGF receptor. Through the phosphorylation of its tyrosine sites, VEGF2 can activate phospholipase C (PLC)-γ, and further downstream protein kinase C. Activated protein kinase C can induce MAPK signaling, which results in endothelial cell proliferation. In addition, VEGF2 induces angiogenesis by means of RAS homolog gene family-guanosine triphosphatase (Rho-GTPase) activation and influences the secretion of proteins like endothelial Von Willebrand Factor, thus increasing endothelial vascular permeability (27-30). VEGF3 on the other hand is thought to play a role in lymphangiogenesis through the activation of protein kinase C and the RAS pathway (27,28). Due to HCC being a highly vascularized tumor, VEGF signaling increases tumor cell growth and proliferation. Data has shown that high levels of VEGF indicate a poor prognosis for patients with HCC (31,32).
Most targeted agents evaluated act on tyrosine kinase receptors involved in growth factor and angiogenic pathways. Select drugs with activity against HCC and their molecular targets are shown in Table 2.
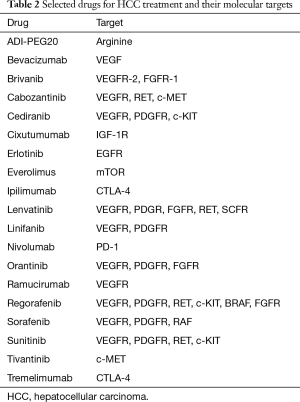
Full table
Completed phase III clinical trials
Inhibitors of angiogenesis
Since the landmark SHARP and Asia Pacific trials demonstrated a survival benefit in advanced HCC in 2008 and 2009, phase III clinical trials evaluating novel agents have thus far yielded negative results. Most drugs target angiogenesis-related pathways, including VEGFR, PDGFR, FGFR, and RET. Of note, almost all trials predominantly included patients with Child-Pugh liver function class A, and were designed to prove non-inferiority. We briefly present select completed phase III trials and summarize their major findings (Table 3).
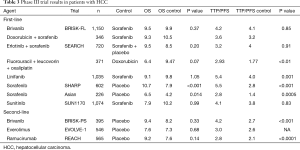
Full table
Sorafenib
Sorafenib was shown to improve survival in multiple randomized controlled trials (RCTs) and is the standard of care for advanced HCC (33,34). The SHARP trial led to sorafenib’s US food and drug administration (FDA) and European Medicines Agency (EMA) approval for the treatment of HCC in 2007 and 2006, respectively, by showing a significant increase in the overall survival (OS) of patients with advanced HCC from 7.9 to 10.7 months (P<0.001). This increased OS was seen in all pre-stratified patient groups, including those with poor performance status and macroscopic vascular invasion or hepatic spread. Time to radiologically observed progression (TTP) was significantly increased with sorafenib compared with placebo (5.5 vs. 2.8 months; P<0.001) while there was a trend toward a longer time to symptomatic progression following placebo (4.9 vs. 4.1 months, P=0.77). The disease control rate was significantly higher with sorafenib (43% vs. 32%; P=0.002) (5).
Sorafenib-related adverse effects were the most common reason for treatment discontinuation in the SHARP study—80% of the treatment group reported adverse effects, which included diarrhea, hand-foot skin reaction, hypertension, and abdominal pain. The most frequent adverse events leading to treatment discontinuation were those affecting the gastrointestinal tract (5).
Between 2005 and 2007, Cheng and colleagues studied the use of sorafenib in advanced HCC for patients in the Asia-Pacific region (6); the study included 271 patients from China, South Korea, and Taiwan. The median OS of patients treated with sorafenib was 6.5 months, compared with 4.2 months for those receiving placebo (P=0.014). The TTP was also significantly longer following sorafenib treatment (2.8 vs. 1.4 months; P=0.0005). The most common adverse effects were hand-foot skin reaction, diarrhea, alopecia, fatigue, rash, and desquamation (6).
Neither the SHARP nor the Asia-Pacific trials stratified patients for etiology of HCC. However, regarding patients with viral hepatitis, the majority of patients in the SHARP trial had HCV whereas the majority of patients in the Asian trial had had HBV. Thus, in the SHARP trial, 60.4% of viral hepatitis patients were infected with HCV and 39.6% were infected with HBV, whereas in the Asian trial, 89.7% of viral hepatitis patients had HBV and only 10.3% had HCV. Because of the worse outcomes noted in the Asian trial, a retrospective analysis was carried out on a phase II trial of HCC patients who received sorafenib. The aim of this post factum analysis was to observe variation in outcomes of patients with HBV and HCV. Forty-six patients were included: 33 with HBV and 13 with HCV. TTP was found to be significantly longer in HCV patients compared to HBV patients (6.5 vs. 4 months, respectively, P=0.05). However, there was no significant improvement in OS or progression-free survival (PFS). Due to the small sample size and the fact that this was a retrospective analysis, it cannot be concluded that sorafenib is more efficacious in HCV patients over HBV patients (35).
Patients who are unable to tolerate, or have tumors that progress on sorafenib therapy are obviously in need of further treatment options. A number of agents have been, or are currently being, investigated as first- and second-line treatment options for advanced HCC.
Brivanib
The dual VEGFR and FGFR inhibitor brivanib has been under investigation in the first-line advanced-stage HCC setting (BRISK-FL trial), in combination with TACE for intermediate stage HCC, and as salvage therapy in patients who failed sorafenib (BRISK-PS trial) (36-38). The BRISK-FL trial, statistically designed for non-inferiority against sorafenib, demonstrated an OS of 9.5 months for brivanib compared with 9.9 months for sorafenib (HR, 1.06; 95% CI, 0.93–1.22; P=0.373). There was also no difference in TTP between brivanib and sorafenib (4.2 months vs. 4.1 months; HR, 1.01; 95% CI, 0.88–1.16; P=0.853). In the BRISK-PS trial, patients treated with brivanib were found to have a median OS of 9.4 months compared with 8.2 months for placebo (HR, 0.89; 95% CI, 0.69–1.15; P=0.331). TTP however, was significantly higher in the brivanib arm compared with placebo (4.2 vs. 2.7 months; HR, 0.56; 95% CI, 0.42–0.78; P<0.001). The trial that investigated brivanib in combination with TACE was terminated early due to the lack of encouraging results from the BRISK trials. Intention to treat analysis of this study indicated no improvement in OS following TACE + brivanib compared with TACE + placebo. Most common grade III/IV adverse effects in all three trials were hypertension, hyponatremia, fatigue, and decreased appetite (38).
Sunitinib
The multikinase inhibitor, sunitinib, targets VEGFR, PDGFR, c-KIT, and RET. The SUN1170 trial compared sunitinib to sorafenib as first-line therapy in advanced HCC (39). Median OS for sunitinib was found to be 7.9 months compared with 10.2 months for sorafenib (HR, 1.30; 95% CI, 1.13–1.50; P=0.0019). TTP in the sunitinib arm was 4.1 vs. 3.8 months in the sorafenib arm (HR, 1.13; P=0.3082). Severe adverse events were significantly worse with sunitinib (thrombocytopenia, 29.7%; and neutropenia, 25.7%). Thus, OS and safety favored sorafenib and the trial was terminated early due to toxicity and futility.
Linifanib
Linifanib is a selective inhibitor of VEGFR and PDGFR. A phase III trial accrued 1,035 patients in a comparison of linifanib and sorafenib as first-line therapy. Trial investigators found no difference in median OS (9.1 vs. 9.8 months; HR, 1.05; 95% CI, 0.90–1.22), although TTP was significantly higher for linifanib (5.4 vs. 4.0 months; HR, 0.76; 95% CI, 0.64–0.90; P=0.001), and ORR was also higher (13% with linifanib and 6.9% with sorafenib) (40). Linifanib was poorly tolerated overall; the agent caused frequent grade 3/4 side effects that required dose interruption and reduction, and/or discontinuation of patients from treatment.
Ramucirumab
Ramucirumab is a recombinant immunoglobulin G1 (IgG1) monoclonal antibody that specifically binds to the extracellular domain of VEGFR-2 with high affinity, thus preventing binding of VEGF ligands and receptor activation. As ramucirumab is an inhibitor of angiogenesis, it was hypothesized that it would be effective in the management of HCC, which is a highly angiogenic malignancy. In a Phase III trial, the REACH study randomized 565 patients with advanced sorafenib-refractory HCC to ramucirumab versus placebo. All patients had an ECOG performance status of 0 to 1 and Child-Pugh A liver disease (patients with Child-Pugh B or C were excluded). Median OS was not significantly different between the two groups (9.2 months for ramucirumab vs. 7.6 months for placebo; HR, 0.866; 95% CI, 0.717–1.046; P=0.1391). Likewise, median PFS was not significantly different (ramucirumab, 2.8 months vs. placebo, 2.1 months). Furthermore, patients receiving ramucirumab had a higher incidence of grade 1 or 2 peripheral edema (36% vs. 18% in placebo), ascites (22% vs. 11%), and headache (18% vs. 5%). Grade 3 or higher adverse events that were found to be at least five percentage-points higher with ramucirumab compared to placebo included ascites, hypertension, asthenia, AST elevation, thrombocytopenia, and hyperbilirubinemia. Thus, ramucirumab is not approved for advanced HCC treatment in a second-line setting. However, alpha-fetoprotein (AFP) levels—known to be a marker of poor prognosis—were monitored in the REACH trial. When patients were stratified into two groups according to baseline AFP levels of greater or less than 400 ng/mL, those with levels of greater than 400 ng/mL who received ramucirumab over placebo had an improved OS of 7.8 vs. 4.2 months, respectively (P=0.006). However, in those patients with an AFP of less than 400 ng/mL, there was no significant difference in outcomes between ramucirumab and placebo groups. This implies that patients with a higher AFP may have a better response to ramucirumab in the second-line setting, a concept that is now being studied in the ongoing REACH-2 trial (41).
Inhibitors of cell proliferation
Everolimus
The EVOLVE-1 study evaluated the mTOR inhibitor everolimus vs. placebo in patients with advanced HCC who had failed sorafenib. There was no significant difference in OS between everolimus (7.6 months) and placebo (7.3 months) (HR, 1.05; 95% CI, 0.86–1.27; P=0.68). TTP in the everolimus arm was 3.0 months compared with 2.6 months in the placebo group (HR, 0.93; 95% CI, 0.75–1.15). The most common grade 3/4 adverse effects caused by everolimus were anemia, asthenia, and decreased appetite (42).
Erlotinib
Erlotinib, a targeted anti-EGFR therapy, was studied in the SEARCH trial in 2015. This study compared erlotinib plus sorafenib with sorafenib alone using a non-inferiority design (43). Median OS was 9.5 months in the sorafenib plus erlotinib arm and 8.5 months in the sorafenib only arm (HR, 0.92; 95% CI, 0.78–1.1; P=0.2). Likewise, TTP was not significantly different between the two groups (3.2 months with sorafenib plus erlotinib vs. 4 months with sorafenib alone; HR, 1.13; 95% CI, 0.94–1.36; P=0.91). Higher rates of rash/desquamation, anorexia, and diarrhea were observed in the sorafenib plus erlotinib group. Furthermore, because of the increased adverse event profile of erlotinib, patients treated with sorafenib plus erlotinib were more frequently discontinued from the trial. Because of these results, erlotinib was not approved for the treatment of patients with advanced HCC (43).
Cytotoxic systemic chemotherapy
Systemic chemotherapy has played a minor role in the treatment of advanced HCC. Recent trials have evaluated chemotherapeutic agents as frontline therapy for this disease. A trial evaluating doxorubicin plus sorafenib vs. doxorubicin alone demonstrated significantly improved TTP and OS in the doxorubicin plus sorafenib treated patient group, compared with doxorubicin alone, but no difference in toxicity (44). TTP was 6.4 months in the sorafenib-doxorubicin group, and 2.8 months in the doxorubicin monotherapy group (P=0.02). Median OS was 13.7 and 6.5 months, respectively (P=0.006). However, initial enthusiasm was hampered following results from a larger trial that enrolled 346 patients and used sorafenib as the control arm, which was terminated early due to futility. A median OS of 9.3 months was observed for doxorubicin plus sorafenib, compared with 10.5 months for sorafenib alone (HR, 1.06; 95% CI, 0.8–1.4); median PFS was 3.6 vs. 3.2 months (HR, 0.90; 95% CI, 0.72–1.2) (45).
Combination chemotherapy with 5-fluorouracil, leucovorin, and oxaliplatin (FOLFOX4) was compared with doxorubicin monotherapy in an Asian trial (46). Median OS was 6.40 months with FOLFOX4 and 4.97 months with doxorubicin (HR, 0.80; 95% CI, 0.63 to 1.02; P=0.07); median PFS was 2.93 vs. 1.77 months (HR, 0.62; 95% CI, 0.49–0.79; P<0.001). Grade 3/4 adverse effects were similar in both groups. Although this trial demonstrated improved OS following FOLFOX4 treatment, sorafenib—the current standard of care for advanced HCC—was not used as a comparison, thus limiting the applicability of these findings (46).
Novel agents and ongoing trials
The landscape of HCC trials has shifted from anti-angiogenic agents to novel drugs that have diverse mechanisms of action. These include agents that target a range of growth factor receptors [e.g., c-MET (receptor for hepatocyte growth factor), KIT (receptor for stem cell growth factor), and RET (neurotropic factor receptor)], agents that target metabolic pathways (e.g., arginine deiminase), and those that target immune checkpoint molecules (PD-1 and CTLA-4). Many of these agents have shown promising data in phase II trials. We present select ongoing trials and interim data.
Regorafenib, a multikinase inhibitor that has antiangiogenic and other activities (47), can be effective when given post-sorafenib treatment. Regorafenib effects its antiangiogenic activity by targeting VEGFR-1-3 and TIE-2. It exerts other anticancer activities by targeting c-KIT, RET, and both wild type and V600 mutated B-RAF, and affecting the tumor microenvironment by targeting PDGFR and FGFR. Sorafenib, similarly, targets VEGFR-1-3, PDGFR, c-KIT, but also targets RAF-MEK-ERK, FLT-3, and RET pathways. An open label phase II study of regorafenib showed an OS of 13.8 months and TTP of 4.3 months in a single cohort of patients with advanced HCC unresponsive to sorafenib (38). The most common regorafenib-related side effects were hand-foot syndrome, fatigue, and anorexia. Most recently, the phase III placebo-controlled RESORCE trial demonstrated significantly improved OS of HCC patients compared with placebo: 10.6 months with regorafenib vs. 7.8 months with placebo (P<0.001) in the second-line setting. This study included 573 patients receiving regorafenib at a dose of 160 mg daily for 3 weeks followed by a 1-week off-treatment period (4-week cycle). Median PFS was 3.1 months following regorafenib compared with 1.5 months following placebo (P<0.001), translating into a 54% reduction in risk of progression or death. The ORR with regorafenib was 10.6%, compared with only 4.1% for placebo (P=0.005). Regorafenib is likely to be approved for the treatment of advanced stage HCC, eight years after the approval of sorafenib (48,49).
Targets in cell proliferation and metastasis
c-MET: tivantinib
Tivantinib is a selective oral inhibitor of c-MET (50). A randomized phase II trial in patients with advanced HCC who failed sorafenib treatment showed a statistically significant improvement in TTP in the Tivantinib group vs. placebo (1.6 vs. 1.4 months; HR 0.64; 90% CI, 0.43–0.94; P=0.04). When selecting for patients with c-MET-high tumors, TTP with tivantinib was nearly twice that of the placebo group (2.7 vs. 1.4 months; HR 0.43; 95% CI, 0.19–0.97; P=0.03). The most common grade 3 adverse event in the tivantinib group was neutropenia (51). A phase III trial (JET-HCC) comparing tivantinib with placebo in patients who failed sorafenib is ongoing. This trial is also examining c-MET expression to further evaluate responsiveness to tivantinib (52).
New angiogenesis inhibitors
Cabozantinib
Cabozantinib is a multiple tyrosine kinase with activity against MET, RET, and VEGFRs, all essential pathways in the development of HCC. A phase II clinical trial evaluated the use of Cabozantinib in patients who were previously treated and failed sorafenib therapy. Median PFS was 4.2 months, and there was 78% measurable disease regression. Most common grade 3 adverse effects were diarrhea, hand foot syndrome, and thrombocytopenia (53). Because of this encouraging data, there is an ongoing phase III trial called CELESTIAL that is evaluating Cabozantinib versus placebo in patients who failed sorafenib (54).
Lenvatinib
Lenvatinib is an inhibitor of VEGFR-1-3, FGFR-1-4, PDGFR-β, RET, and KIT. A phase I/II study showed a median TTP of 12.8 months (95% CI, 7.23–14.7) and an OS of 18.7 months (95% CI, 12.8–25.1). The most common adverse events were hypertension, hand foot syndrome, proteinuria, anorexia, thrombocytopenia, and fatigue (55). In a currently running phase III RCT, lenvatinib is being compared to sorafenib in the frontline treatment of patients with advanced HCC (55).
New anti-metabolites
ADI-PEG20
Arginine deiminase is a microbial enzyme that depletes arginine when injected systemically. Early studies showed that arginine deprivation inhibited HCC growth in vitro but normal liver tissue survives arginine depletion (56). A phase II study looked at 71 patients with advanced HCC and randomly assigned them to weekly ADI-PEG 20 at doses of 150 or 320 IU m2. Of these patients, 46.3% had failed previous systemic therapy. There were no objective responses. Each dose group had 11 patients with stable disease. The median duration of disease control was 2.8 months for both dose cohorts with a 95% CI of 1.4–4.3 months in the 160 IU m2 cohort and 2.4–3.3 months in the 320 IU m2 cohort. There was no statistically significant difference in OS between the two dose groups: 6.2 months in the 320 IU m2 arm and 8.4 months in the 160 IU m2 arm, P=0.76. OS for all patients was 7.3 months. Most common treatment-related adverse events were systemic and local allergic reactions and metabolic changes of grade 1–2: hypersensitivity skin rash in 26.8% of patients, local tissue reaction at injection site in 22.5%, hyperuricemia in 19.7%, pruritus in 15.5%, fatigue in 9.8%, and hyperammonemia in 4.2% (57). Because of the good toxicity profile and the data on HCC requiring arginine for cell growth and proliferation, ADI-PEG20 was further studied in HCC. A phase III trial evaluating the use of ADI-PEG20 in patients who failed first-line treatment was completed and showed that there was no OS benefit in the population receiving ADI-PEG 20 compared with placebo. Six hundred and thirty five patients were enrolled and randomized 2:1 to ADI-PEG20, at a dose of 18 mg/m2 IM weekly, or placebo, both with best supportive care. Results showed that the median OS for ADI-PEG 20 was 7.8 vs. 7.4 months for placebo (P=0.884; not significant). Median PFS was also not significantly different: both groups had a PFS of 2.6 months (P=0.075). However, there was a significant increase in OS benefit in patients with prolonged arginine depletion. Patients with arginine depletion for more than 8 weeks had a median OS of 12.3 months compared with 7.3 months in patients who had arginine depletion for four weeks or less (P=0.0032). Patients who received ADI-PEG 20 had a higher rate of anaphylaxis (2.1%). Fifteen percent of patients receiving ADI-PEG20 died within 20 days of last dose compared with 10.4% of patients receiving placebo (58).
More potent pure anti-angiogenic inhibitors
Cediranib
Cediranib (AZD2171) is an oral inhibitor of VEGFR. In 2012, a phase II trial was carried out by the North Central Cancer Treatment Group, which administered daily cediranib, at a dose of 45 mg or less, to 28 patients with unresectable or metastatic HCC. The primary study objective was to achieve an OS of 6 months. Patients had an ECOG performance status of 0 or 1 and had received no previous systemic therapy or external beam radiation to their primary tumor site. At 6 months, 12 patients (42.9%) were still alive, 15 patients (53.6%) had died, and one (3.5%) was lost to follow-up. Twelve of the 15 patients who died had no radiologic evidence of progression before death. During treatment, a total of 7 patients had stable disease, 7 developed progression of disease, and 14 were not evaluated post-baseline. The median TTP was 2.8 months and median OS was 5.8 months (95% CI: 3.4–7.3 months). No partial or complete responses were observed. Cediranib was poorly tolerated: grade 4 adverse effects included valvular heart disease, anemia, dehydration, liver infection, transaminitis, and fatigue (59).
In 2013, Zhu et al. (60) looked at the efficacy, pharmacokinetics, and possible biomarkers of cediranib monotherapy in a phase II study. As shown in Table 2, cediranib and sorafenib both inhibit VEGFR and PDGFR; however cediranib differs from sorafenib in that it does not have activity against RAF. In this phase II study, cediranib was given at a dose of 30 mg daily to patients with unresectable or metastatic HCC. Biomarkers—VEGF, PlGF, sVEGFR-1, bFGF, IL-6, IL-8, TNF-alpha, IFN-gamma, HGF, IGF-1, sVEGFR-2, angiopoietin-2, c-KIT, CAIX, SDF1-alpha, sTie2—were measured before and after treatment. Seventeen patients were enrolled with a median follow-up time of 17 months. The median PFS was 5.3 months (95% CI, 3.5–9.7 months) and the median OS was 11.7 months (95% CI, 7.5–13.6 months). The 3-month PFS rate was 77%. Fewer adverse effects were observed than with higher doses of cediranib. Even so, 5% of patients had grade 3 fatigue, and 29% had grade 3 hypertension that was medically manageable. Hyponatremia and hyperbilirubinemia were commonly observed (65% and 18% of patients, respectively). Biomarker studies indicated that that PlGF and VEGF concentrations were increased, and sVEGFR-1, sVEGFR-2, and Ang-2 were decreased by cediranib. sVEGFR-1 and -2 were linked to poor survival and there was an association between high pretreatment levels of VEGF (or CAIX and Ang-2) levels and rapid progression, indicating tumor hypoxia is a poor prognostic marker for HCC. Proangiogenic factors, bFGF and IGF-1, were also correlated with poor treatment outcomes. IFN- gamma levels were associated with a longer PFS (60).
Immune checkpoint inhibitors
Nivolumab + ipilimumab
The interaction between lymphocytes and HCC cells is regulated by checkpoint inhibitor proteins such as PD-1 and CTLA-4, which induce immune tolerance upon binding to their respective ligands. One study demonstrated overexpression of PD-L1 in HCC cells. An ex vivo study using extracted cytotoxic CD8+ T lymphocytes from HCC patients demonstrated increased immunogenicity against selected tumor antigens after exposure to the CTLA-4 inhibitor, ipilimumab (61,62). This provided the rationale for a phase I/II trial evaluating the efficacy of immunotherapy against HCC. The trial started with a dose escalation followed by an expansion phase, aimed at establishing a safe dose of nivolumab in three separate cohorts by disease etiology (HCC patients with HBV, HCV, or no co-infection) and assessing nivolumab efficacy in these three separate patient groups. The third part of the study is assessing the efficacy of nivolumab plus sorafenib combined, and the fourth part of the study is assessing the safety and efficacy of nivolumab plus ipilimumab combined in the treatment of advanced HCC. Preliminary results showed that nivolumab yielded good response rates in patients with advanced HCC. Forty-two patients were enrolled in the first phases and 19% had greater than 30% tumor reduction, half of which continued to respond for 12 months. The OS rate was 62% at 12 months (63).
Tremelimumab
Tremelimumab is a CTLA-4 inhibitor that potentiates T-cell activation and proliferation against several solid tumors. A pilot study evaluated the use of tremelimumab in 20 patients with advanced HCC and HCV infection Patients were given tremelimumab at a dose of 15 mg/kg IV every 90 days until disease progression or severe toxicity. Partial response rate was 17.6% and disease control rate was 76.4%. TTP was 6.48 months (95% CI, 3.95–9.14) and a significant drop in viral load was observed. The most common grade III side effect was liver function test elevation (64).
Conclusions
Since the landmark SHARP trial in 2007, sorafenib monotherapy remains the only widely accepted standard treatment for advanced HCC. While newer agents have failed to demonstrate significantly improved survival in clinical trials, our understanding of the tumor biology and novel biomarkers continues to expand. However, this knowledge-gain has not yet translated into development of groundbreaking treatments. In a recent study by Llovet et al., exploring the reasons for trial failure, the authors identified four key obstacles to success during the development of new therapies for HCC: clinical translation of genomic studies, faulty trial design, liver toxicity, and marginal efficacy of tested agents. There is a low prevalence of molecular targets in HCC upon which most developed agents act. The past decade has seen multiple negative trials, many of which have not been guided by our understanding of genetic pathways in HCC. Current efforts should be directed toward the development of biomarker-enriched clinical trials, as well as drugs that target key oncogenic pathways with a better understanding of the tumor biology and genetics (65-67).
Acknowledgments
We would like to thank Marion Hartley, PhD, Science Writer for Clinical Research at the Ruesch Center, Lombardi, for her edits and suggestions during the composition of this review.
Footnote
Conflicts of Interest: The authors have no conflicts of interest to declare.
References
- Torre LA, Bray F, Siegel RL, et al. Global cancer statistics, 2012. CA Cancer J Clin 2015;65:87-108. [Crossref] [PubMed]
- Yeh CN, Chen MF, Lee WC, et al. Prognostic factors of hepatic resection for hepatocellular carcinoma with cirrhosis: univariate and multivariate analysis. J Surg Oncol 2002;81:195-202. [Crossref] [PubMed]
- Bupathi M, Kaseb A, Meric-Bernstam F, et al. Hepatocellular carcinoma: Where there is unmet need. Mol Oncol 2015;9:1501-9. [Crossref] [PubMed]
- Lammer J, Malagari K, Vogl T, et al. Prospective randomized study of doxorubicin-eluting-bead embolization in the treatment of hepatocellular carcinoma: results of the PRECISION V study. Cardiovasc Intervent Radiol 2010;33:41-52. [Crossref] [PubMed]
- Llovet JM, Ricci S, Mazzaferro V, et al. Sorafenib in advanced hepatocellular carcinoma. N Engl J Med 2008;359:378-90. [Crossref] [PubMed]
- Cheng AL, Kang YK, Chen Z, et al. Efficacy and safety of sorafenib in patients in the Asia-Pacific region with advanced hepatocellular carcinoma: a phase III randomised, double-blind, placebo-controlled trial. Lancet Oncol 2009;10:25-34. [Crossref] [PubMed]
- Llovet JM, Villanueva A, Lachenmayer A, et al. Advances in targeted therapies for hepatocellular carcinoma in the genomic era. Nat Rev Clin Oncol 2015;12:436. [Crossref] [PubMed]
- Guichard C, Amaddeo G, Imbeaud S, et al. Integrated analysis of somatic mutations and focal copy-number changes identifies key genes and pathways in hepatocellular carcinoma. Nat Genet 2012;44:694-8. [Crossref] [PubMed]
- Kan Z, Zheng H, Liu X, et al. Whole-genome sequencing identifies recurrent mutations in hepatocellular carcinoma. Genome Res 2013;23:1422-33. [Crossref] [PubMed]
- Totoki Y, Tatsuno K, Covington KR, et al. Trans-ancestry mutational landscape of hepatocellular carcinoma genomes. Nat Genet 2014;46:1267-73. [Crossref] [PubMed]
- Farazi PA, DePinho RA. Hepatocellular carcinoma pathogenesis: from genes to environment. Nat Rev Cancer 2006;6:674-87. [Crossref] [PubMed]
- Gao Q, Wang XY, Qiu SJ, et al. Overexpression of PD-L1 significantly associates with tumor aggressiveness and postoperative recurrence in human hepatocellular carcinoma. Clin Cancer Res 2009;15:971-9. [Crossref] [PubMed]
- Schulze K, Imbeaud S, Letouzé E, et al. Exome sequencing of hepatocellular carcinomas identifies new mutational signatures and potential therapeutic targets. Nat Genet 2015;47:505-11. [Crossref] [PubMed]
- Klaus A, Birchmeier W. Wnt signalling and its impact on development and cancer. Nat Rev Cancer 2008;8:387-98. [Crossref] [PubMed]
- Takigawa Y, Brown AM. Wnt signaling in liver cancer. Curr Drug Targets 2008;9:1013-24. [Crossref] [PubMed]
- Wong CM, Fan ST, Ng IO. beta-Catenin mutation and overexpression in hepatocellular carcinoma: clinicopathologic and prognostic significance. Cancer 2001;92:136-45. [Crossref] [PubMed]
- Paez J, Sellers WR. PI3K/PTEN/AKT pathway. A critical mediator of oncogenic signaling. Cancer Treat Res 2003;115:145-67. [Crossref] [PubMed]
- Shin JW, Chung YH. Molecular targeted therapy for hepatocellular carcinoma: current and future. World J Gastroenterol 2013;19:6144-55. [Crossref] [PubMed]
- Organ SL, Tsao MS. An overview of the c-MET signaling pathway. Ther Adv Med Oncol 2011;3:S7-S19. [Crossref] [PubMed]
- Graziani A, Gramaglia D. Hepatocyte growth factor/scatter factor stimulates the Ras-guanine nucleotide exchanger. J Biol Chem 1993;268:9165-8. [PubMed]
- Ridley AJ, Comoglio PM, Hall A. Regulation of scatter factor/hepatocyte growth factor responses by Ras, Rac, and Rho in MDCK cells. Mol Cell Biol 1995;15:1110-22. [Crossref] [PubMed]
- Trusolino L, Bertotti A, Comoglio PM. MET signalling: principles and functions in development, organ regeneration and cancer. Nat Rev Mol Cell Biol 2010;11:834-48. [Crossref] [PubMed]
- Peruzzi B, Bottaro DP. Targeting the c-Met signaling pathway in cancer. Clin Cancer Res 2006;12:3657-60. [Crossref] [PubMed]
- You H, Ding W, Dang H, et al. c-Met represents a potential therapeutic target for personalized treatment in hepatocellular carcinoma. Hepatology 2011;54:879-89. [Crossref] [PubMed]
- Goyal L, Muzumdar MD, Zhu AX. Targeting the HGF/c-MET pathway in hepatocellular carcinoma. Clin Cancer Res 2013;19:2310-8. [Crossref] [PubMed]
- Cancer incidence in five continents. Volume IX. IARC Sci Publ 2008.1-837. [PubMed]
- Kaseb AO, Hanbali A, Cotant M, et al. Vascular endothelial growth factor in the management of hepatocellular carcinoma: a review of literature. Cancer 2009;115:4895-906. [Crossref] [PubMed]
- Shibuya M. Vascular Endothelial Growth Factor (VEGF) and Its Receptor (VEGFR) Signaling in Angiogenesis: A Crucial Target for Anti- and Pro-Angiogenic Therapies. Genes Cancer 2011;2:1097-105. [Crossref] [PubMed]
- Roskoski R Jr. Vascular endothelial growth factor (VEGF) signaling in tumor progression. Crit Rev Oncol Hematol 2007;62:179-213. [Crossref] [PubMed]
- Neufeld G, Cohen T, Gengrinovitch S, et al. Vascular endothelial growth factor (VEGF) and its receptors. FASEB J 1999;13:9-22. [PubMed]
- Mihaylova MM, Shaw RJ. The AMPK signalling pathway coordinates cell growth, autophagy and metabolism. Nat Cell Biol 2011;13:1016-23. [Crossref] [PubMed]
- Zhan P, Qian Q, Yu LK. Serum VEGF level is associated with the outcome of patients with hepatocellular carcinoma: a meta-analysis. Hepatobiliary Surg Nutr 2013;2:209-15. [PubMed]
- Peng S, Zhao Y, Xu F, et al. An updated meta-analysis of randomized controlled trials assessing the effect of sorafenib in advanced hepatocellular carcinoma. PLoS One 2014;9:e112530. [Crossref] [PubMed]
- Kudo M, Imanaka K, Chida N, et al. Phase III study of sorafenib after transarterial chemoembolisation in Japanese and Korean patients with unresectable hepatocellular carcinoma. Eur J Cancer 2011;47:2117-27. [Crossref] [PubMed]
- Huitzil FD, Saltz LS, Song J, et al. Retrospective analysis of outcome in hepatocellular carcinoma (HCC) patients (pts) with hepatitis C (C+) versus B (B+) treated with sorafenib (S). Proc Am Soc Clin Oncol 2011;29:abstr e14636.
- Johnson PJ, Qin S, Park JW, et al. Brivanib versus sorafenib as first-line therapy in patients with unresectable, advanced hepatocellular carcinoma: results from the randomized phase III BRISK-FL study. J Clin Oncol 2013;31:3517-24. [Crossref] [PubMed]
- Kudo M, Han G, Finn RS, et al. Brivanib as adjuvant therapy to transarterial chemoembolization in patients with hepatocellular carcinoma: A randomized phase III trial. Hepatology 2014;60:1697-707. [Crossref] [PubMed]
- Llovet JM, Decaens T, Raoul JL, et al. Brivanib in patients with advanced hepatocellular carcinoma who were intolerant to sorafenib or for whom sorafenib failed: results from the randomized phase III BRISK-PS study. J Clin Oncol 2013;31:3509-16. [Crossref] [PubMed]
- Cheng AL, Kang YK, Lin DY, et al. Sunitinib versus sorafenib in advanced hepatocellular cancer: results of a randomized phase III trial. J Clin Oncol 2013;31:4067-75. [Crossref] [PubMed]
- Cainap C, Qin S, Huang WT, et al. Linifanib versus Sorafenib in patients with advanced hepatocellular carcinoma: results of a randomized phase III trial. J Clin Oncol 2015;33:172-9. [Crossref] [PubMed]
- Zhu AX, Park JO, Ryoo BY, et al. Ramucirumab versus placebo as second-line treatment in patients with advanced hepatocellular carcinoma following first-line therapy with sorafenib (REACH): a randomised, double-blind, multicentre, phase 3 trial. Lancet Oncol 2015;16:859-70. [Crossref] [PubMed]
- Zhu AX, Kudo M, Assenat E, et al. Effect of everolimus on survival in advanced hepatocellular carcinoma after failure of sorafenib: the EVOLVE-1 randomized clinical trial. JAMA 2014;312:57-67. [Crossref] [PubMed]
- Zhu AX, Rosmorduc O, Evans TR, et al. SEARCH: a phase III, randomized, double-blind, placebo-controlled trial of sorafenib plus erlotinib in patients with advanced hepatocellular carcinoma. J Clin Oncol 2015;33:559-66. [Crossref] [PubMed]
- Abou-Alfa GK, Johnson P, Knox JJ, et al. Doxorubicin plus sorafenib vs doxorubicin alone in patients with advanced hepatocellular carcinoma: a randomized trial. JAMA 2010;304:2154-60. [Crossref] [PubMed]
- Abou-Alfa GK, Niedzwieski D, Knox JJ, et al. Phase III randomized study of sorafenib plus doxorubicin versus sorafenib in patients with advanced hepatocellular carcinoma (HCC): CALGB 80802 (Alliance). J Clin Oncol 2016;34:abstr 192.
- Qin S, Bai Y, Lim HY, et al. Randomized, multicenter, open-label study of oxaliplatin plus fluorouracil/leucovorin versus doxorubicin as palliative chemotherapy in patients with advanced hepatocellular carcinoma from Asia. J Clin Oncol 2013;31:3501-8. [Crossref] [PubMed]
- Wilhelm SM, Dumas J, Adnane L, et al. Regorafenib (BAY 73-4506): a new oral multikinase inhibitor of angiogenic, stromal and oncogenic receptor tyrosine kinases with potent preclinical antitumor activity. Int J Cancer 2011;129:245-55. [Crossref] [PubMed]
- Bruix J, Tak WY, Gasbarrini A, et al. Regorafenib as second-line therapy for intermediate or advanced hepatocellular carcinoma: multicentre, open-label, phase II safety study. Eur J Cancer 2013;49:3412-9. [Crossref] [PubMed]
- Bruix J, Finn RS, Kudo M, et al. RESORCE: An ongoing randomized, double-blind, phase III trial of regorafenib (REG) in patients with hepatocellular carcinoma (HCC) progressing on sorafenib (SOR). J Clin Oncol 2014;32:abstr TPS4156^.
- Adjei AA, Schwartz B, Garmey E. Early clinical development of ARQ 197, a selective, non-ATP-competitive inhibitor targeting MET tyrosine kinase for the treatment of advanced cancers. Oncologist 2011;16:788-99. [Crossref] [PubMed]
- Santoro A, Rimassa L, Borbath I, et al. Tivantinib for second-line treatment of advanced hepatocellular carcinoma: a randomised, placebo-controlled phase 2 study. Lancet Oncol 2013;14:55-63. [Crossref] [PubMed]
- Kyowa Hakko Kirin Company. A randomized double-blind, placebo-controlled Japanese phase III trial of ARQ 197 in hepatocellular carcinoma (HCC) (JET-HCC). In: ClinicalTrials.gov (Internet). Bethesda (MD). National Library of Medicine (US). 2000-(cited 2016 Nov 1) (NCT02029157). (Recruiting).
- Verslype C, Cohn AL, Kelley RK, et al. Activity of cabozantinib (XL184) in hepatocellular carcinoma: Results from a phase II randomized discontinuation trial (RDT). J Clin Oncol 2012;30:abstr 4007.
- Exelixis. Study of Cabozantinib (XL184) vs placebo in subjects with hepatocellular carcinoma who have received prior sorafenib (CELESTIAL). In: ClinicalTrials.gov (Internet). (NCT01908426) (This study is ongoing, but not recruiting participants).
- Finn RS, Cheng AL, Ikeda K, et al. A multicenter, open-label, phase 3 trial to compare the efficacy and safety of lenvatinib (E7080) versus sorafenib in first-line treatment of subjects with unresectable hepatocellular carcinoma. J Clin Oncol 2014;32:abstr TPS4153.
- Ensor CM, Holtsberg FW, Bomalaski JS, et al. Pegylated arginine deiminase (ADI-SS PEG20,000 mw) inhibits human melanomas and hepatocellular carcinomas in vitro and in vivo. Cancer Res 2002;62:5443-50. [PubMed]
- Yang TS, Lu SN, Chao Y, et al. A randomised phase II study of pegylated arginine deiminase (ADI-PEG 20) in Asian advanced hepatocellular carcinoma patients. Br J Cancer 2010;103:954-60. [Crossref] [PubMed]
- Abou-Alfa GK, Qin S, Ryoo BY, et al. Phase III randomized study of second line ADI-peg 20 (A) plus best supportive care versus placebo (P) plus best supportive care in patients (pts) with advanced hepatocellular carcinoma (HCC). J Clin Oncol 2016;34:abstr 4017.
- Alberts SR, Fitch TR, Kim GP, et al. Cediranib (AZD2171) in patients with advanced hepatocellular carcinoma: a phase II North Central Cancer Treatment Group Clinical Trial. Am J Clin Oncol 2012;35:329-33. [Crossref] [PubMed]
- Zhu AX, Ancukiewicz M, Supko JG, et al. Efficacy, safety, pharmacokinetics, and biomarkers of cediranib monotherapy in advanced hepatocellular carcinoma: a phase II study. Clin Cancer Res 2013;19:1557-66. [Crossref] [PubMed]
- Mizukoshi E, Nakamoto Y, Arai K, et al. Comparative analysis of various tumor-associated antigen-specific t-cell responses in patients with hepatocellular carcinoma. Hepatology 2011;53:1206-16. [Crossref] [PubMed]
- El-Khoueiry AB, Melero I, Crocenzi TS, et al. Phase I/II safety and antitumor activity of nivolumab in patients with advanced hepatocellular carcinoma (HCC): CA209-040. J Clin Oncol 2015;33:abstr LBA101.
- Bristol-Myers Squibb. A study to evaluate the effectiveness, safety and tolerability of nivolumab and the combination nivolumab plus ipilimumab in patients with advanced liver cancer (CheckMate040). In: ClinicalTrials.gov (Internet). Bethesda (MD). National Library of Medicine (US). 2000-(cited 2016 Nov 1) (NCT01658878) (This study is currently recruiting participants).
- Sangro B, Gomez-Martin C, de la Mata M, et al. A clinical trial of CTLA-4 blockade with tremelimumab in patients with hepatocellular carcinoma and chronic hepatitis C. J Hepatol 2013;59:81-8. [Crossref] [PubMed]
- Sun X, Sui Q, Zhang C, et al. Targeting blockage of STAT3 in hepatocellular carcinoma cells augments NK cell functions via reverse hepatocellular carcinoma-induced immune suppression. Mol Cancer Ther 2013;12:2885-96. [Crossref] [PubMed]
- Llovet JM, Hernandez-Gea V. Hepatocellular carcinoma: reasons for phase III failure and novel perspectives on trial design. Clin Cancer Res 2014;20:2072-9. [Crossref] [PubMed]
- Hoshida Y, Nijman SM, Kobayashi M, et al. Integrative transcriptome analysis reveals common molecular subclasses of human hepatocellular carcinoma. Cancer Res 2009;69:7385-92. [Crossref] [PubMed]