NCAPG is transcriptionally regulated by CBX3 and activates the Wnt/β-catenin signaling pathway to promote proliferation and the cell cycle and inhibit apoptosis in colorectal cancer
Highlight box
Key findings
• NCAPG might help to impede the progression of CRC.
What is known and what is new?
• NCAPG transcriptionally regulated by CBX3 activated the Wnt/β-catenin signaling pathway to promote proliferation and cell cycle in HCT116 cells.
• NCAPG transcriptionally regulated by CBX3 activated the Wnt/β-catenin signaling pathway to inhibit apoptosis in HCT116 cells.
What is the implication, and what should change now?
• NCAPG might be a therapeutic target for the amelioration of CRC.
Introduction
Colorectal cancer (CRC) develops from the uncontrolled division or abnormal growth of colon or rectum cells (1). As the second most common adult cancer in females and the third most common in males, CRC is the fourth major contributor to cancer deaths, accounting for 9.2% of deaths worldwide (2,3). In 2020, about 1.5 million people were diagnosed with CRC and more than 50,000 people died of it (4). It is well-documented that environmental and genetic factors are critical in the pathogenesis of CRC (1). In recent years, tremendous progresses have been achieved in surgical and medical treatments, and the potential of targeted therapies has shown great promise (5). Nevertheless, CRC is prone to tumor metastasis and distant metastasis is a critical cause of poor prognosis (6). Many studies have found that individualized targeted therapy is effective for the treatment of tumor metastasis. For example, FEM1C upregulation might inhibit the metastasis in CRC (7). Kang and co-workers have evidenced that the downregulation of miR-138 helped to impede the progression of CRC (8). In view of this, the exploration of independent biomarkers involved in the advancement of CRC is of great significance.
Non-structural maintenance of chromosomes (SMC) condensin I complex subunit G (NCAPG) is a mitosis-associated chromosomal condensing protein (9). It was reported that high expression of NCAPG correlated with immune cell infiltration in the tumor microenvironment (9,10). As a tumor-promoting gene, NCAPG is overexpressed in some malignant tumors, including castration‐resistant prostate cancer and hepatocellular carcinoma (11,12). Interestingly, Shi and co-workers demonstrated that NCAPG expression was upregulated in CRC tissues and cell lines and the knockdown of NCAPG exhibited inhibitory effects on the proliferation, migration, and invasion of HCT116 and SW480 cells (13). Besides, the overexpression of NCAPG was evidenced to serve as a candidate biomarker for CRC prognosis (14). Nevertheless, the biological function of NCAPG in CRC still requires further investigation.
Chromobox protein homolog 3 (CBX3) is a member of the heterochromatin-associated protein 1 (HP1) family (15). By inhibiting the expression level of a variety of genes, CBX3 acts as a critical regulator in many cellular processes, such as cell growth, differentiation, and DNA damage (16). Aberrant expression of CBX3 has been found to be implicated in the progression of various cancer types, including gastric cancer and glioma (17,18). More importantly, CBX3 has been shown to be upregulated in colon cancer cells and to promote cell cycle progression and proliferation (19). In addition, according to the Human Transcription Factor Database (HumanTFDB; http://bioinfo.life.hust.edu.cn/HumanTFDB#!/), CBX3 could bind to NCAPG promoters, while data from the Encyclopedia of RNA Interactomes (ENCORI) database (https://starbase.sysu.edu.cn/) revealed that CBX3 was positively correlated with NCAPG.
Wnt/β-catenin signaling has been widely implicated in many human cancers, including gastric cancer (20), breast cancer (21), and prostate cancer (22), and CRC is no exception (23,24). As a core factor of the Wnt signaling pathway, β-catenin is a bridge of the Wnt signaling pathway with epithelial-mesenchymal transformation (EMT) (13). Studies have testified that EMT is a primary cause of the recurrence and metastasis of cancer because it can increase the migration and invasion of cancer cells and plays a critical role in the modulation of CRC diagnosis and prognosis (25,26). The trigger of Wnt/β-catenin signaling has been demonstrated to be a vital player in EMT required for CRC metastasis (27). In addition, NCAPG has been shown to activate Wnt/β-catenin signaling in many diseases. For example, NCAPG overexpression suppressed apoptosis and promoted EMT in cardia adenocarcinoma via triggering Wnt/β-catenin signaling (28). Elsewhere, NCAPG facilitated the progression of CRC and EMT process via activation of Wnt/β-catenin signaling (13).
This study was undertaken to investigate the functional role of NCAPG in CRC and to explore its mechanism, aiming to provide a theoretical foundation for further studies. We present the following article in accordance with the MDAR reporting checklist (available at https://jgo.amegroups.com/article/view/10.21037/jgo-23-63/rc).
Methods
Cell culture and treatment
Normal colon mucosal epithelial cell line NCM460 was supplied from Biovector (Beijing, China) while CRC cell lines (HCT116, SW480, and HT-29) were provided by Procell Life Science & Technology Co., Ltd. (Wuhan, China). All cells were cultivated in Dulbecco’s modified Eagle’s medium (DMEM, Biosharp Life Sciences, Hefei, China), supplemented with 10% fetal bovine serum (FBS, Thermo Fisher Scientific Inc., Waltham, MA, USA) and 1% penicillin-streptomycin (Sigma-Aldrich, St. Louis, MO, USA), at 37 ℃ with 5% CO2.
Cell transfection
HCT116 cells were harvested in the logarithmic growth phase and then injected into 6-well plates at a density of 1×105 cells/mL. Plasmids carrying CBX3 (Ov-CBX3), pcDNA3.1 empty vector (Ov-NC), short hairpin specific to CBX3 (sh-CBX3) and NCAPG (sh-NCAPG), and the corresponding scrambled sequence as a negative control (sh-NC) were synthesized by Obio Technology Co., Ltd. (Shanghai, China). The transfection of 100 nM of recombinants into HCT116 cells was performed at 37 ℃ for 48 hours using Lipofectamine 2000 reagent (Thermo Fisher Scientific Inc.) After 48 hours, the cells were collected for subsequent experiments.
Reverse transcription-quantitative polymerase chain reaction (RT-qPCR)
RNA was extracted from HCT116 cells with TRIzol reagent (Biosharp) according to the manufacturer’s instructions. Complementary DNA (cDNA) was synthesized using the PrimeScript RT Reagent Kit with genomic DNA (gDNA) Eraser (Takara Bio Inc., Kusatsu, Shiga, Japan). Subsequently, PCR amplification was conducted with TB Green Premix Ex Taq II on the ABI PRISM 7900 Sequence Detection System (Applied Biosystems) in line with the suggested recommendations. The following thermocycling conditions were used: initial denaturation at 95 ℃ for 5 minutes, followed by 40 cycles of denaturation at 95 ℃ for 10 seconds and annealing at 60 ℃ for 30 seconds, and then extension for 10 minutes at 72 ℃. The primer sequences were as follows: NCAPG forward (F), 5'-GAGGCTGCTGTCGATTAAGGA-3' and reverse (R), 5'-AACTGTCTTATCATCCATCGTGC-3', CBX3 F, 5'-TAGATCGACGTGTAGTGAATGGG-3' and R, 5'-TGTCTGTGGCACCAATTATTCTT-3' and β-actin F, 5'-AGCGAGCATCCCCCAAAGTT-3' and R, 5'-GGGCACGAAGGCTCATCATT-3'. The relative gene expression was determined with 2−ΔΔCT (29).
Western blot
HCT116 cells were harvested in the logarithmic growth phase and then injected into 6-well plates at a density of 1×105 cells/mL. Total proteins were extracted from HCT116 cells with radioimmunoprecipitation assay (RIPA) lysis buffer (Biosharp) and then quantified with the application of a bicinchoninic acid (BCA) protein assay kit (Biosharp). After separation with 10% sodium dodecyl sulphate-polyacrylamide gel electrophoresis (SDS-PAGE), equal amounts of protein (30 µg/lane) were transferred onto polyvinylidene difluoride (PVDF) membranes (Millipore, Burlington, MA, USA). Membranes were blocked with 5% bovine serum albumin (BSA, BioFroxx) at room temperature for 1 hour. The membranes were then cultivated with primary antibodies targeting NCAPG (24563-1-AP; 1:5,000; Proteintech, Wuhan, China), cyclin D1 (60186-1-AP; 1:5,000; Proteintech), cyclin-dependent kinase 4 (CDK4) (66950-1-Ig; 1:5,000; Proteintech), Bcl2 (12789-1-AP; 1:2,000; Proteintech), Bax (50599-2-Ig; 1:2,000; Proteintech), CBX3 (ab217999; 1:2,000; Abcam), Wnt3a (26744-1-AP; 1:500; Proteintech), β-catenin (66379-1-Ig; 1:5,000; Proteintech) or β‑actin (20536-1-AP; 1:2,000; Proteintech) overnight at 4 ℃. Next, the membranes were rinsed with tris-buffered saline with Tween (TBST) and then incubated with horseradish peroxidase (HRP)-conjugated affinipure goat anti-mouse IgG (SA00001-1; 1:2,000; Proteintech) or HRP-conjugated affinipure goat anti-rabbit IgG (SA00001-2; 1:2,000; Proteintech) at 37 ℃ for 2 h. The antibody-labeled proteins were visualized using enhanced chemiluminescence (ECL) detection reagent (Shanghai Yeasen Biotechnology Co., Ltd., Shanghai, China) and then analyzed using ImageJ, version 1.49 (National Institutes of Health, USA).
Cell Counting Kit-8 (CCK-8) assay
HCT116 cells harvested in the logarithmic growth phase were injected into 96-well plates at a density of 3×104 cells/mL and then cultivated at room temperature for 24 hours. Afterwards, 15 µL CCK-8 solution was added into each well to further incubate HCT116 cells in the dark at 37 ℃ with 5% CO2 for 2 hours. The optical density was determined utilizing a microplate reader (Thermo Fisher Scientific Inc.) at 450 nm.
Flow cytometry
HCT116 cells harvested in the logarithmic growth phase were injected into 6-well plates at a density of 1×105 cells/mL and incubated at room temperature. After centrifugation at 1,500 r/min for 5 minutes, the cell precipitate was collected and the supernatant was discarded. Subsequently, the collected precipitate was rinsed with pre-chilled PBS and subjected to 75% ethanol fixation at 4 ℃ overnight. The cells were then exposed to 100 µL RNase A (Sigma-Aldrich) at 37 ℃ for 30 minutes and stained with 400 µL propidium iodide (Sigma-Aldrich) at 4 ℃ away from light for 30 minutes. Finally, the cells were analyzed using a flow cytometer (Beckman Coulter, Brea, CA, USA).
Terminal-deoxynucleotidyl transferase dUTP nick-end labeling (TUNEL) assay
The apoptosis of HCT116 cells was determined using a TUNEL kit (Beyotime Institute of Biotechnology, Shanghai, China). HCT116 cells harvested in the logarithmic growth phase were injected into 48-well plates at a density of 1×104 cells/mL and then treated as aforementioned. Subsequently, HCT116 cells were subjected to 4% paraformaldehyde (Beyotime Institute of Biotechnology) fixation at room temperature for 30 minutes and 0.25% Triton X-100 (Beyotime Institute of Biotechnology) permeation at room temperature for 5 minutes. Next, HCT116 cells were rinsed with phosphate-buffered saline (PBS) twice and then incubated with 50 µL reaction solution for 1 hour as per the standard protocol, after which 0.1 µg/mL 4',6-diamidino-2-phenylindole (DAPI) was applied for the staining of cell nuclei at room temperature for 10 minutes. Finally, apoptotic cells in 5 randomly selected fields were photographed under a florescent microscope (Olympus, Tokyo, Japan).
Colorimetric caspase activity assay
HCT116 cells harvested in the logarithmic growth phase were injected into 6-well plates at a density of 1×105 cells/mL for incubation. According to manufacturer’s instructions, the activity of caspase3 and caspase9 was assessed with caspase3 assay kit and caspase9 assay kit (Elabscience, Houston, TX, USA), respectively. The optical density was determined at 405 nm with a microplate reader (Thermo Fisher Scientific Inc.).
Luciferase report assay
HumanTFDB predicted the binding sites of CBX3 and NCAPG promoters. Luciferase assay was implemented for the verification of their interaction on the Luciferase Reporter System (Promega, Madison, WI, USA). NCAPG wild-type (WT) as well as mutant (MUT) reporter plasmids containing CBX3 mimic or mimic NC binding sites were constructed (GenePharma, Shanghai, China) to be transfected into HCT116 cells with Lipofectamine 2000 reagent (Thermo Fisher Scientific Inc.) as per standard protocol. Luciferase activity was normalized to that of Renilla.
Statistical analysis
All experiments were repeated at least 3 times. The collected experimental data are presented in the form of mean ± standard deviation (SD) and were analyzed with GraphPad Prism 8.0 software (GraphPad Software Inc., San Diego, CA, USA). One-way analysis of variance (ANOVA) with Tukey’s post-hoc test was applied for comparisons among multiple groups. P less than 0.05 indicated statistical significance.
Results
NCAPG silence inhibited the proliferation and cell cycle of HCT116 cells
The messenger RNA (mRNA) and protein expressions of NCAPG in normal colon mucosal epithelial cell line NCM460 and CRC cell lines (HCT116, SW480, and HT-29) were first assessed using RT-qPCR and western blot. Compared with the NCM460 group, the mRNA and protein expressions of NCAPG were greatly elevated in CRC cell lines (Figure 1A,1B). It was noted that NCAPG had higher expression in HCT116 cells, and thus they were chosen for subsequent experiments. To reduce NCAPG expression, sh-NCAPG was transfected into HCT116 cells, and RT-qPCR as well as western blot was utilized to examine transfection efficacy. Compared with the sh-NC group, the expression of NCAPG was the lowest in the sh-NCAPG#3 group, and thus sh-NCAPG#3 was selected for following studies (Figure 1C,1D). CCK-8 was conducted to determine the effects of NCAPG knockdown on the proliferation of HCT116 cells. As depicted in Figure 1E, the proliferation of HCT116 cells was conspicuously reduced after depleting NCAPG expression when compared with the sh-NC group. In addition, results obtained from flow cytometry showed that NCAPG deficiency markedly decreased the number of HCT116 cells in the S phase of the cell cycle in comparison with the sh-NC group (Figure 1F). Moreover, the expressions of cell cycle-related proteins were analyzed using western blot, which showed that the expressions of cyclin D1 and CDK4 were markedly decreased in NCAPG-silenced HCT116 cells (Figure 1G). The above results indicated that NCAPG depletion exhibited inhibitory effects on the proliferation and cell cycle of HCT116 cells.
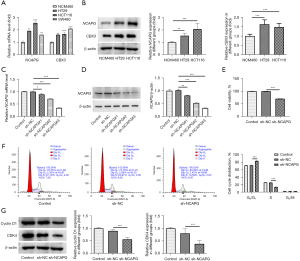
NCAPG silence induced the apoptosis of HCT116 cells
The effects of NCAPG silence on the apoptosis of HCT116 cells were evaluated with TUNEL. As demonstrated in Figure 2A, the apoptosis level of HCT116 cells was conspicuously enhanced after silencing NCAPG expression in contrast with the sh-NC group. The contents of apoptosis-related proteins were determined with western blot, which revealed that NCAPG knockdown elevated Bax expression and decreased Bcl2 expression compared with those in the sh-NC group (Figure 2B). In comparison with the sh-NC group, the activity of cleaved caspase3 in HCT116 cells was markedly enhanced after cell transfection with sh-NCAPG (Figure 2C). To conclude, the apoptosis of HCT116 cells could be induced by silencing NCAPG.
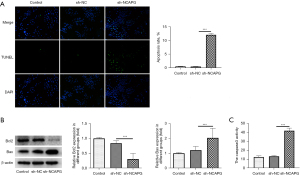
NCAPG is transcriptionally regulated by CBX3
HumanTFDB predicted the binding sites of CBX3 and NCAPG promoters (Table 1 and Figure 3A). According to the ENCORI database, CBX3 was positively correlated with NCAPG (Figure 3B). The mRNA and protein expressions of CBX3 were assessed by RT-qPCR and western blot. The results showed that the mRNA and protein expressions of CBX3 were markedly increased in CRC cell lines (HCT116, SW480, and HT-29) when compared to NCM460 cells (Figure 1A,1B). To overexpress CBX3, Ov-CBX3 was transfected into HCT116 cells, which led to a significant elevation of the mRNA and protein expressions of CBX3 in HCT116 cells. Sh-CBX3 was also transfected into HCT116 cells, and the results showed that the expression of CBX3 in HCT116 cells was conspicuously decreased compared with the sh-NC group (Figure 3C,3D). Evidently, sh-CBX3#2 had better transfection efficacy, as shown by the lower expression of CBX3 in the sh-CBX3#2 group. In view of this, sh-CBX3#2 was selected for subsequent experiments. Results obtained from luciferase report assay revealed that conspicuously stronger luciferase activity was observed in HCT116 cells transfected with Ov-CBX3 and NCAPG WT than that in HCT116 cells transfected with Ov-NC and NCAPG WT (Figure 3E). Compared with Ov-NC, the expression of NCAPG in CBX3-overexpressed HCT116 cells was greatly increased, while the expression of NCAPG in CBX3-silenced HCT116 cells was reduced compared with the sh-NC group (Figure 3F,3G). To sum up, NCAPG was transcriptionally regulated by CBX3.
Table 1
TF | Site | Start | Stop | Score | P value | Matched sequence |
---|---|---|---|---|---|---|
CBX3 | Site 3 | 581 | 592 | 12.9605 | 1.77E-05 | CAGAAAATCCAT |
CBX3 | Site 4 | 797 | 818 | 11.6282 | 2.85E-05 | ACGCAGAGGAGTCCCACCATGG |
CBX3 | Site 2 | 234 | 249 | 11.2632 | 2.94E-05 | TACAATGGAGTACTAT |
CBX3 | Site 5 | 1,738 | 1,746 | 11.3947 | 3.02E-05 | CAGCCAGGC |
CBX3 | Site 1 | 122 | 133 | 10.6184 | 6.16E-05 | CACAAAGCAAAA |
CBX3, chromobox protein homolog 3; NCAPG, non-SMC condensin I complex subunit G; SMC, structural maintenance of chromosomes; HumanTFDB, Human Transcription Factor Database; TF, transcription factor.
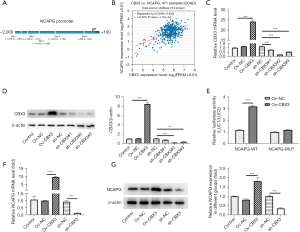
NCAPG transcriptionally regulated by CBX3 activated the Wnt/β-catenin signaling pathway to regulate proliferation, the cell cycle, and apoptosis in HCT116 cells
To determine the relationship between CBX3 and the Wnt/β-catenin signaling pathway, western blot was performed to evaluate the contents of Wnt/β-catenin signaling pathway-related proteins. Compared with the Ov-NC group, CBX3 overexpression elevated the expressions of Wnt3a and β-catenin in HCT116 cells, indicating that CBX3 could activate the Wnt/β-catenin signaling pathway (Figure 4A). Results obtained from CCK-8 demonstrated that the reduced viability in HCT116 cells due to NCAPG depletion was partially revived after overexpressing CBX3 (Figure 4B). Compared with the sh-NC group, NCAPG deficiency increased the number of cells in the G0/G1 phase and decreased that in the S phase, which was then reversed by CBX3 overexpression (Figure 4C). Additionally, the reduced protein expressions of cyclin D1 and CDK4 in NCAPG-silenced HCT116 cells were elevated after cell transfection with Ov-CBX3 compared with the sh-NCAPG + Ov-NC group (Figure 4D). Cell apoptosis was determined with TUNEL, and the results showed that NCAPG knockdown induced apoptosis in HCT116 cells compared with the sh-NC, while CBX3 overexpression exhibited the opposite effect, as indicated by diminished apoptosis in the sh-NCAPG + Ov-CBX3 group (Figure 4E). Compared with the sh-NC group, NCAPG interference increased Bax expression and reduced Bcl2 expression, which were subsequently reversed by CBX3 overexpression (Figure 4F). Further, CBX3 overexpression also suppressed the enhanced activity of cleaved caspase3 and cleaved caspase9 in NCAPG-depleted HCT116 cells in comparison with the sh-NCAPG + Ov-NC group (Figure 4G). Collectively, our results showed that NCAPG was transcriptionally regulated by CBX3 and activated the Wnt/β-catenin signaling pathway to promote proliferation and the cell cycle and inhibit apoptosis in HCT116 cells.
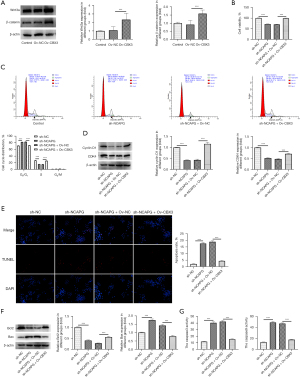
Discussion
CRC is one of the most common cancers worldwide (30). Due to tumor heterogeneity, significant differences in phenotypic features such as proliferation and apoptosis are often exhibited during the onset and in the aggressiveness of CRC (31). As is commonly known, abnormal proliferation is one of the characteristics of cancer cells (32). As a critical regulator of cancer progression, the cell cycle has become an emerging target for treatment in breast cancer (33). Further, dysregulated apoptotic cell death is a hallmark for cancer because the changes in apoptosis are closely related with tumor development (34). In view of this, it can be concluded that the interruption of the above processes is an effective way to protect against cancer development. Besides, previous studies have found many important biomarkers in the progression of CRC and claimed that molecular targeted-therapy is critical for the treatment of CRC (35,36). For instance, long noncoding RNA SNHG14 was confirmed to facilitate CRC progression (37). Additionally, IMPDH2 was also showed to promote the malignant development of CRC cells (38). NCAPG is a condensin complex subunit encoded by the NY-MEL-3 gene and is upregulated in CRC (13). In addition, NCAPG has been found to promote proliferation and suppress apoptosis in CRC cells (39), and NCAPG is involved in cell cycle progression (40). In the present study, RT-qPCR and western blot showed that the mRNA and protein expressions of NCAPG were upregulated in CRC cells (HCT116, HT-29, and SW480). After transfection with sh-NCAPG, the expression of NCAPG was greatly reduced. Moreover, NCAPG knockdown was found to repress the proliferation. The cell cycle in CRC cells was also repressed by NCAPG interference, accompanied by decreased protein expressions of cyclin D1 and CDK4. However, NCAPG deficiency induced apoptosis in CRC cells, together with increased Bax expression and reduced Bcl2 expression. The above findings implied that NCAPG knockdown helped to fight against CRC.
CBX3 has a close association with transcriptional activation or repression, cell differentiation and growth, as well as epigenetic modification (19,41). Previous study has reported that CBX3 is elevated in many cancers and is crucial for the advancement of tumors and the modulation of growth-related genes (42). For instance, CBX3 was highly expressed in osteosarcoma and predictive of an unfavorable prognosis for osteosarcoma patients (43). Peng et al. reported that CBX3 expression was enhanced in glioblastoma (GBM) and promoted the proliferation, invasion, and tumorigenesis of GBM cells (44). Moreover, CBX3 was shown to be positively expressed in CRC tissues (45). In this study, we found that the mRNA and protein expressions of CBX3 were conspicuously elevated in CRC cells, which was consistent with the findings of a previous study (45). After transfection with Ov-CBX3, the expression of CBX3 in HCT116 cells was highly expressed, while sh-CBX3 reduced CBX3 expression. HumanTFDB predicted the binding sites of CBX3 and NCAPG promoters, and according to the ENCORI database, CBX3 was positively correlated with NCAPG. Here, results obtained from luciferase report assay demonstrated that the luciferase activity in HCT116 cells transfected with Ov-CBX3 and NCAPG WT was higher than that in HCT116 cells transfected with Ov-NC and NCAPG WT. Additionally, Ov-CBX3 elevated the expression of NCAPG while sh-CBX3 decreased that in HCT116 cells, indicating that NCAPG could be regulated by CBX3.
The aberrant Wnt/β-catenin signaling pathway has been shown to promote cancer stem cell renewal as well as cell proliferation, thereby acting as a critical player in tumorigenesis and therapy response (46). A number of studies have highlighted the therapeutic potential of agents targeting Wnt/β-catenin signaling in cancer (47), including colon cancer (48). Mukherjee et al. reported that targeting Wnt/β-catenin signaling was an effective method in the treatment of breast cancer (21). Interestingly, Disoma and co-workers put forward that Wnt/β-catenin signaling should be a pivotal therapeutic target for CRC (49). In the present study, the expressions of Wnt/β-catenin signaling-related proteins (Wnt3a and β-catenin) were determined, and the results showed that the contents of Wnt3a and β-catenin were enhanced in CBX3-overexpressed HCT116 cells, implying that CBX3 could activate Wnt/β-catenin signaling in CRC. To investigate the mechanism of Wnt/β-catenin signaling, further experiments were conducted, with the results showing that the effects of NCAPG knockdown on the proliferation, cell cycle, and apoptosis on HCT116 cells were reversed by CBX3 overexpression.
Conclusions
To sum up, this study investigated the functional role of NCAPG in HCT116 cells and identified that NCAPG could be transcriptionally regulated by CBX3, revealing the mechanism through which the progression of CRC is facilitated by NCAPG. To the best our knowledge, this study was the first to explore the mechanism of NCAPG and CBX3 in CRC, which shed novel insights into the targeted treatment for CRC and laid foundation for further researches.
Acknowledgments
Funding: This work was supported by Collaborative Innovation Center of Chinese Ministry of Education (No. 2020-39).
Footnote
Reporting Checklist: The authors have completed the MDAR reporting checklist. Available at https://jgo.amegroups.com/article/view/10.21037/jgo-23-63/rc
Data Sharing Statement: Available at https://jgo.amegroups.com/article/view/10.21037/jgo-23-63/dss
Conflicts of Interest: All authors have completed the ICMJE uniform disclosure form (available at https://jgo.amegroups.com/article/view/10.21037/jgo-23-63/coif). The authors have no conflicts of interest to declare.
Ethical Statement: The authors are accountable for all aspects of the work in ensuring that questions related to the accuracy or integrity of any part of the work are appropriately investigated and resolved.
Open Access Statement: This is an Open Access article distributed in accordance with the Creative Commons Attribution-NonCommercial-NoDerivs 4.0 International License (CC BY-NC-ND 4.0), which permits the non-commercial replication and distribution of the article with the strict proviso that no changes or edits are made and the original work is properly cited (including links to both the formal publication through the relevant DOI and the license). See: https://creativecommons.org/licenses/by-nc-nd/4.0/.
References
- Ebrahimzadeh S, Ahangari H, Soleimanian A, et al. Colorectal cancer treatment using bacteria: focus on molecular mechanisms. BMC Microbiol 2021;21:218. [Crossref] [PubMed]
- Bray F, Ferlay J, Soerjomataram I, et al. Global cancer statistics 2018: GLOBOCAN estimates of incidence and mortality worldwide for 36 cancers in 185 countries. CA Cancer J Clin 2018;68:394-424. [Crossref] [PubMed]
- Dekker E, Tanis PJ, Vleugels JLA, et al. Colorectal cancer. Lancet 2019;394:1467-80. [Crossref] [PubMed]
- Siegel RL, Miller KD, Goding Sauer A, et al. Colorectal cancer statistics, 2020. CA Cancer J Clin 2020;70:145-64. [Crossref] [PubMed]
- Rosen AW, Degett TH, Gögenur I. Individualized treatment of colon cancer. Ugeskr Laeger 2016;178:V11150916. [PubMed]
- Wang J, Li S, Liu Y, et al. Metastatic patterns and survival outcomes in patients with stage IV colon cancer: A population-based analysis. Cancer Med 2020;9:361-73. [Crossref] [PubMed]
- Huang R, Li J, Fu Y, et al. Downregulation of FEM1C enhances metastasis and proliferation in colorectal cancer. Ann Transl Med 2021;9:1391. [Crossref] [PubMed]
- Kang X, Kong B, Chen Q, et al. Low expression of miR-138 inhibit the proliferation, migration and invasion of colorectal cancer and affect patient survival by targeting SIRT1. Transl Cancer Res 2021;10:3548-59. [Crossref] [PubMed]
- Jiang X, Shi Y, Chen X, et al. NCAPG as a Novel Prognostic Biomarker in Glioma. Front Oncol 2022;12:831438. [Crossref] [PubMed]
- Zhou Y, Fan Y, Mao Y, et al. NCAPG is a prognostic biomarker of immune infiltration in non-small-cell lung cancer. Biomark Med 2022;16:523-35. [Crossref] [PubMed]
- Arai T, Okato A, Yamada Y, et al. Regulation of NCAPG by miR-99a-3p (passenger strand) inhibits cancer cell aggressiveness and is involved in CRPC. Cancer Med 2018;7:1988-2002. [Crossref] [PubMed]
- Liu W, Liang B, Liu H, et al. Overexpression of non-SMC condensin I complex subunit G serves as a promising prognostic marker and therapeutic target for hepatocellular carcinoma. Int J Mol Med 2017;40:731-8. [Crossref] [PubMed]
- Shi Y, Ge C, Fang D, et al. NCAPG facilitates colorectal cancer cell proliferation, migration, invasion and epithelial-mesenchymal transition by activating the Wnt/β-catenin signaling pathway. Cancer Cell Int 2022;22:119. [Crossref] [PubMed]
- Sun DP, Wu CC, Chou CL, et al. NCAPG deregulation indicates poor patient survival and contributes to colorectal carcinogenesis. Pathol Res Pract 2023;241:154238. [Crossref] [PubMed]
- Oyama K, El-Nachef D, Fang C, et al. Deletion of HP1γ in cardiac myocytes affects H4K20me3 levels but does not impact cardiac growth. Epigenetics Chromatin 2018;11:18. [Crossref] [PubMed]
- Jin X, Zhang B, Zhang H, et al. Smoking-associated upregulation of CBX3 suppresses ARHGAP24 expression to activate Rac1 signaling and promote tumor progression in lung adenocarcinoma. Oncogene 2022;41:538-49. [Crossref] [PubMed]
- Lin H, Lian J, Xia L, et al. CBX3 Promotes Gastric Cancer Progression and Affects Factors Related to Immunotherapeutic Responses. Cancer Manag Res 2020;12:10113-25. [Crossref] [PubMed]
- Zhao SP, Wang F, Yang M, et al. CBX3 promotes glioma U87 cell proliferation and predicts an unfavorable prognosis. J Neurooncol 2019;145:35-48. [Crossref] [PubMed]
- Fan Y, Li H, Liang X, et al. CBX3 promotes colon cancer cell proliferation by CDK6 kinase-independent function during cell cycle. Oncotarget 2017;8:19934-46. [Crossref] [PubMed]
- Yu Z, Jiang X, Qin L, et al. A novel UBE2T inhibitor suppresses Wnt/β-catenin signaling hyperactivation and gastric cancer progression by blocking RACK1 ubiquitination. Oncogene 2021;40:1027-42. Erratum in: Oncogene 2021;40:2622-3. [Crossref] [PubMed]
- Mukherjee N, Panda CK. Wnt/β-Catenin Signaling Pathway as Chemotherapeutic Target in Breast Cancer: An Update on Pros and Cons. Clin Breast Cancer 2020;20:361-70. [Crossref] [PubMed]
- Schneider JA, Logan SK. Revisiting the role of Wnt/β-catenin signaling in prostate cancer. Mol Cell Endocrinol 2018;462:3-8. [Crossref] [PubMed]
- Bian J, Dannappel M, Wan C, et al. Transcriptional Regulation of Wnt/β-Catenin Pathway in Colorectal Cancer. Cells 2020;9:2125. [Crossref] [PubMed]
- Cheng X, Xu X, Chen D, et al. Therapeutic potential of targeting the Wnt/β-catenin signaling pathway in colorectal cancer. Biomed Pharmacother 2019;110:473-81. [Crossref] [PubMed]
- Nieszporek A, Skrzypek K, Adamek G, et al. Molecular mechanisms of epithelial to mesenchymal transition in tumor metastasis. Acta Biochim Pol 2019;66:509-20. [Crossref] [PubMed]
- Zhu S, Zhao G, Zhao X, et al. Elevated soluble E-cadherin during the epithelial-mesenchymal transition process and as a diagnostic marker in colorectal cancer. Gene 2020;754:144899. [Crossref] [PubMed]
- Cai W, Ye Q, She QB. Loss of 4E-BP1 function induces EMT and promotes cancer cell migration and invasion via cap-dependent translational activation of snail. Oncotarget 2014;5:6015-27. [Crossref] [PubMed]
- Zhang X, Zhu M, Wang H, et al. Overexpression of NCAPG inhibits cardia adenocarcinoma apoptosis and promotes epithelial-mesenchymal transition through the Wnt/β-catenin signaling pathway. Gene 2021;766:145163. [Crossref] [PubMed]
- Livak KJ, Schmittgen TD. Analysis of relative gene expression data using real-time quantitative PCR and the 2(-Delta Delta C(T)) Method. Methods 2001;25:402-8. [Crossref] [PubMed]
- Lulli M, Napoli C, Landini I, et al. Role of Non-Coding RNAs in Colorectal Cancer: Focus on Long Non-Coding RNAs. Int J Mol Sci 2022;23:13431. [Crossref] [PubMed]
- Yang YL, Chu JY, Wang MR. Tumor genetic heterogeneity. Yi Chuan 2013;35:1-9. [Crossref] [PubMed]
- Lu W, Zhang H, Niu Y, et al. Long non-coding RNA linc00673 regulated non-small cell lung cancer proliferation, migration, invasion and epithelial mesenchymal transition by sponging miR-150-5p. Mol Cancer 2017;16:118. [Crossref] [PubMed]
- Koirala N, Dey N, Aske J, et al. Targeting Cell Cycle Progression in HER2+ Breast Cancer: An Emerging Treatment Opportunity. Int J Mol Sci 2022;23:6547. [Crossref] [PubMed]
- Pistritto G, Trisciuoglio D, Ceci C, et al. Apoptosis as anticancer mechanism: function and dysfunction of its modulators and targeted therapeutic strategies. Aging (Albany NY) 2016;8:603-19. [Crossref] [PubMed]
- Mangiapane LR, Nicotra A, Turdo A, et al. PI3K-driven HER2 expression is a potential therapeutic target in colorectal cancer stem cells. Gut 2022;71:119-28. [Crossref] [PubMed]
- Berlin C, Cottard F, Willmann D, et al. KMT9 Controls Stemness and Growth of Colorectal Cancer. Cancer Res 2022;82:210-20. [Crossref] [PubMed]
- Di W, Weinan X, Xin L, et al. Long noncoding RNA SNHG14 facilitates colorectal cancer metastasis through targeting EZH2-regulated EPHA7. Cell Death Dis 2019;10:514. [Crossref] [PubMed]
- Duan S, Huang W, Liu X, et al. IMPDH2 promotes colorectal cancer progression through activation of the PI3K/AKT/mTOR and PI3K/AKT/FOXO1 signaling pathways. J Exp Clin Cancer Res 2018;37:304. [Crossref] [PubMed]
- Li P, Wen J, Ren X, et al. MicroRNA-23b-3p targets non-SMC condensing I complex subunit G to promote proliferation and inhibit apoptosis of colorectal cancer cells via regulation of the PI3K/AKT signaling pathway. Oncol Lett 2021;22:812. [Crossref] [PubMed]
- Zhang X, Wang H, Han Y, et al. NCAPG Induces Cell Proliferation in Cardia Adenocarcinoma via PI3K/AKT Signaling Pathway. Onco Targets Ther 2020;13:11315-26. [Crossref] [PubMed]
- Canzio D, Larson A, Narlikar GJ. Mechanisms of functional promiscuity by HP1 proteins. Trends Cell Biol 2014;24:377-86. [Crossref] [PubMed]
- Chen LY, Cheng CS, Qu C, et al. CBX3 promotes proliferation and regulates glycolysis via suppressing FBP1 in pancreatic cancer. Biochem Biophys Res Commun 2018;500:691-7. [Crossref] [PubMed]
- Ma C, Nie XG, Wang YL, et al. CBX3 predicts an unfavorable prognosis and promotes tumorigenesis in osteosarcoma. Mol Med Rep 2019;19:4205-12. [Crossref] [PubMed]
- Peng W, Shi S, Zhong J, et al. CBX3 accelerates the malignant progression of glioblastoma multiforme by stabilizing EGFR expression. Oncogene 2022;41:3051-63. [Crossref] [PubMed]
- Wang H, Zhao W, Wang J, et al. Clinicopathological significance of CBX3 in colorectal cancer: An intensive expression study based on formalin-fixed and paraffin-embedded tissues. Pathol Int 2022;72:107-16. [Crossref] [PubMed]
- Zhang Y, Wang X. Targeting the Wnt/β-catenin signaling pathway in cancer. J Hematol Oncol 2020;13:165. [Crossref] [PubMed]
- Yu F, Yu C, Li F, et al. Wnt/β-catenin signaling in cancers and targeted therapies. Signal Transduct Target Ther 2021;6:307. [Crossref] [PubMed]
- Wang J, Cai H, Liu Q, et al. Cinobufacini Inhibits Colon Cancer Invasion and Metastasis via Suppressing Wnt/β-Catenin Signaling Pathway and EMT. Am J Chin Med 2020;48:703-18. [Crossref] [PubMed]
- Disoma C, Zhou Y, Li S, et al. Wnt/β-catenin signaling in colorectal cancer: Is therapeutic targeting even possible? Biochimie 2022;195:39-53. [Crossref] [PubMed]
(English Language Editor: A. Muijlwijk)