Estrogen receptor 1 inhibits the progression of hepatocellular carcinoma via positively regulating lncRNA maternally expressed gene 3 under high glucose conditions
Introduction
With changing lifestyles and increasing ageing of the population, the incidence rate of diabetes mellitus (DM) gets higher year by year, anticipated to rise to 642 million by 2040 worldwide (1,2). Emerging evidence has shown that DM is not only a risk factor for cancer, but also increases cancer mortality (3). Hepatocellular carcinoma (HCC) is the most common cancer of the digestive system and the third leading cause of cancer death worldwide (4,5). Recent studies have shown that the incidence of liver cancer is markedly increased in patients with DM compared to patients without DM (6,7). Hyperglycemia may affect the progression and prognosis of HCC by influencing inflammation and promoting insulin resistance (8,9).
Long noncoding RNAs (lncRNAs), one of the RNA transcripts, are emerging as critical regulators in tumor biology, such as cell proliferation, migration, invasion, drug resistance and other physiological processes (10-12). Among various lncRNAs, the roles of a few in HCC have been confirmed, such as Hottip and HULC (13,14). Our previous study showed that lncRNA MEG3 is involved in both DM and tumor development (15). The lncRNA maternally expressed gene 3 (MEG3), a cancer suppressor gene, inhibits the proliferation, migration and invasion of tumor cells (16,17). Studies have shown that lncRNAs share transcription factors (TFs) with protein-coding genes, and the promoters are conserved (18-21). Expression levels of TFs and promoter binding strength regulate lncRNA expression (22). Estrogen receptor 1 (ESR1), one of the ER family of ligand-activated TFs, plays important roles in metabolism, growth, sexual development and other reproductive functions. The receptor encoded by this gene plays a key role in the development of many kinds of cancers and metabolic diseases (23-27). Accordingly, the purpose of this study was to investigate whether the ESR1–lncRNA MEG3 pathway can affect glucose-induced proliferation, migration and invasion of HepG2 cells. We present the following article in accordance with the MDAR reporting checklist (available at https://jgo.amegroups.com/article/view/10.21037/jgo-22-825/rc).
Methods
Bioinformatics analysis
The promoter sequence of lncRNA MEG3 was obtained using the UCSC database (http://genome.ucsc.edu/). The PROMO and JASPAR databases were used for predicting potential TFs. The study was conducted in accordance with the Declaration of Helsinki (as revised in 2013).
Cell culture
Human liver cancer cells (HepG2) were provided by the Cell Bank of the Chinese Academy of Sciences (Shanghai, China). Cells were cultured in DMEM (Gibco, Grand Island, NY, USA) containing 10% fetal bovine serum (FBS, Thermo Fisher Scientific, USA), and incubated at 37 ℃ in a humidified 5% CO2 atmosphere. HepG2 cells were divided into a control group [DMEM with 5.5 mmol/L glucose (LG)] and a high glucose group [DMEM with 25 mmol/L glucose (HG)].
Cell transfection
The small interfering RNAs (siRNAs) targeting ESR1 and siRNA negative control (si-NC), the ESR1-pcDNA3.1 plasmid and vector plasmid (GenePharma, Shanghai, China) were generated. Table 1 shows the siRNA sequences. HepG2 cells were transfected with these plasmids through a Lipofectamine 2000 system (Thermo Fisher Scientific, USA).
Table 1
Gene | Sequence |
---|---|
ESR1-Homo-1014 | 5’-GAGGGAGAAUGUUGAAACATT-3’ |
5’-UGUUUCAACAUUCUCCCUCTT-3’ | |
ESR1-Homo-1380 | 5’-GGCUAGAGAUCCUGAUGAUTT-3’ |
5’-AUCAUCAGGAUCUCUAGCCTT-3’ | |
ESR1-Homo-1533 | 5’-GGUUCCGCAUGAUGAAUCUTT-3’ |
5’-AGAUUCAUCAUGCGGAACCTT-3’ | |
ESR1-siRNA NC | 5’-GGCUACGUCCAGGAGCGCACC-3’ |
5’-UGCGCUCCUGGACGUAGCCUU-3’ |
Total RNA preparation and quantitative real-time polymerase chain reaction (qRT-PCR)
Total RNA was extracted from HepG2 cells and frozen tissue specimens (30–40 mg) by TRIzol (Invitrogen, Carlsbad, CA, USA). The tissues were pulverised by a tissue grinder (SCIENTZ, Ningbo, China), extraction was performed with chloroform and isopropanol, and after the RNA pellet dried, it was dissolved in DEPC water. The RNA concentration was analysed by a Pan-wavelength microplate reader (BioTek Instruments, USA). Next, cDNA was synthesized by a reverse transcription kit (Takara, Beijing, China). Table 2 shows the primer sequences. qRT-PCR reactions were performed with the SYBR Green Master (Takara). The 2-ΔΔCt method was used to calculate the fold change differences in gene expression.
Table 2
Gene | Sequence |
---|---|
ESR1 | 5'-ATGGTCAGTGCCTTGTTGGATGC-3' |
5'-GTCTGCCAGGTTGGTCAGTAAGC-3' | |
ELK1 | 5'-GCCACATCATCTCCTGGACTTCAC-3' |
5'-TGTTGGTCTTGTTCTTGCGTAGCC-3' | |
CREB1 | 5'-CACCTGCCATCACCACTGTAACG-3' |
5'-AGTGGCTGCTGCATTGGTCATG-3' | |
Lnc MEG3 | 5'-CCTTCCATGCTGAGCTGCT-3' |
5'-TGTTGGTGGGATCCAGGAAA-3' | |
GAPDH | 5'-ACCCACTCCTCCACCTTTGAC-3' |
5'-TGTTGCTGTAGCCAAATTCGTT-3' |
qRT-PCR, quantitative real time- polymerase chain reaction.
Luciferase reporter assays
The UCSC website (http://genome.ucsc.edu/) was used to predict the binding sites between ESR1 and lncRNA MEG3. The sequences of wild-type (WT) and mutated (MUT) lncRNA MEG3 promoter were synthesized and inserted into pGL3 vectors for constructing the corresponding reporters. Subsequently, these recombinant reporters were cotransfected with pcDNA3.1/ESR1 or pcDNA3.1 into HepG2 cells. After 48 h of transfection, a dual-luciferase detection kit was used to evaluate the luciferase activity using a dual luciferase reporter analysis system (Promega). Renilla luciferase expression vector pRL-TK (Takara, Beijing, China) was used as an internal control.
RNA immunoprecipitation (RIP) assay
The RIP assay was executed through a Magna RIP RNA-Binding Protein Immunoprecipitation kit (Millipore, Billerica, MA, USA). In detail, cells were lysed in RIP buffer, followed by incubation with magnetic beads conjugated with anti-IgG and anti-Ago2. The immunoprecipitated RNAs were extracted and examined by qRT-PCR to affirm the enrichment of the binding targets.
Cell viability assay
Cell Counting Kit-8 (CCK-8) assay kits (Solarbio, Beijing, China) were utilized for assessing cell viability. HepG2 cells were put in 96-well plates and cultured for 12–72 h, followed by the addition of 10 µL CCK-8 solution. After incubation for 1–2 h, a microplate reader was used to measure the absorbance at 450 nm.
Flow cytometry analysis
Cell apoptosis was evaluated through a fluorescein isothiocyanate (FITC)-conjugated Annexin V and propidium iodide (PI) kit (BD Biosciences, San Diego, CA, USA). In detail, HepG2 cells were washed using phosphate-buffered saline and then stained using Annexin V-FITC and PI for 30 min in the dark. Apoptotic cells were examined via flow cytometry and data were analyzed via FlowJo software (Tree Star, Ashland, OR, USA).
5-ethynyl-2’-deoxyuridine (EdU) assay
After cell transfection, HepG2 cells were inoculated into 96-well plates. An EdU Apollo-567 Kit (RIBOBIO, Shanghai, China) was used to evaluate the change in cell proliferation ability caused by overexpression of ESR1 or silencing of lncRNA MEG3. EdU and DAPI dyes were utilized to treat HepG2 cells, followed by cell visualization using a fluorescent microscope (Olympus, Tokyo, Japan).
Colony formation assay
Cells were mixed with top agar (DMEM, 20% FBS, 0.3% soft agar) and inoculated into 30-mm plates that were coated with 0.5 mL soft agar mixture (0.6% soft agar) on the base. After 2 weeks’ incubation, cells were fixed with methanol, and then stained with 0.1% crystal violet. The number of colonies was counted using an inverted microscope (Olympus).
Transwell assay
Cells (2×105 cells/chamber) were suspended in FBS-free DMEM and placed in the upper compartment of an apical chamber that was coated with Matrigel (8 µm pore-size, Corning Costar, USA). For the migration assay, the chamber was not coated with Matrigel. As a chemoattractant, 700 µL DMEM with 10% FBS were added to the lower compartment of the chamber. After incubation for 24 h, the non-invading cells were removed using a cotton swab. The invading cells were fixed with methanol for 30 min and stained with 0.1% crystal violet for 20 min. An inverted microscope was utilized for quantification in five random visual fields.
Wound healing assay
A wound healing assay was used to assess the migratory ability of HepG2 cells. On the backs of 6-well plates, a perpendicular pen was used to draw a line per 1.0 cm. After cells reached 70–90% confluence, a scratch was made along the marked line. Next, the cells were incubated at 37 ℃ and an inverted microscope was used to obtain images at 0 and 24 h. The wound width was used to assess cell migration. The average scratch width was calculated through ImageJ software.
Western blot analysis
For obtaining proteins, tissues and cells were exposed to RIPA lysis buffer (Beyotime, Shanghai, China) and a protease inhibitor cocktail (Boster, Wuhan, China). Immunoblotting analysis was performed according to standard using primary antibodies containing ESR1 (A12976; Abclonal, Wuhan, China) and β-actin (AC026; Abclonal). Samples of each protein were separated by 8–12% SDS denatured polyacrylamide gel and then transferred onto a nitrocellulose (NC) membrane. The membranes were blocked for 1 h by 5% skim milk and incubated with antibody at 4 ℃ overnight. Next, the membranes were incubated with goat anti-rabbit IgG (H+L)-HRP (Sungene, Tianjin, China) as the secondary antibody (dilution, 1:3,000–5,000). The Immobilon Western Chemiluminescent HRP Substrate (Thermo Fisher Scientific, USA) was used for visualizing protein bands. ImageJ was used for analysis.
Statistical analysis
All experiments were repeated three times. Statistical analyses were performed using SPSS version 22.0 (IBM, New York, USA) and Prism version 8.0 (GraphPad Software, USA). Data are displayed as mean ± SD. Normal distribution and homogeneity test of variance were used for testing data. One-way ANOVA was performed to compare differences of multiple groups. P<0.05 was regarded as statistical significance.
Results
ESR1 identified by bioinformatics
We identified the promoter sequence of lncRNA MEG3 in UCSC (http://genome.ucsc.edu/). Five potential TFs, including ESR1, cAMP responsive element binding protein 1 (CREB1), ETS transcription factor ELK1 (ELK1) CREB1, GATA-binding protein 1 (GATA1), and E26 avian leukemia oncogene 1, 5' domain (ETS1) were predicted by bioinformatics (PROMO, JASPAR) (Figure 1A). ESR1, CREB1, and ELK1 were screened as candidate proteins for subsequent analysis according to the GEPIA database (Table 3), NCBI searching and literature reviewing. Based on the results of qRT-PCR and western blot, we found that the expression level of ESR1 in HG markedly decreased at 48 h compared to LG but the difference disappeared at 24 h (Figure 1B-1E). Concurrently, the mRNA expression of CREB1 and ELK1 was not consistent at 24 and 48 h in HG compared to LG (Figure 1C,1D). According to these results and literature review, ESR1 was selected as the target protein for conducting further analysis.
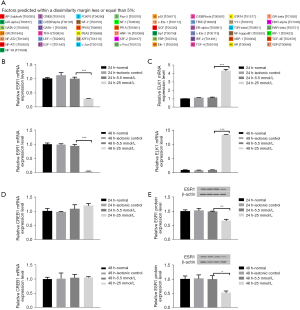
Table 3
TF | HCC tissue | Adjacent tissue |
---|---|---|
CREB1 | 3.52 | 2.86 |
GATA1 | 0 | 0.04 |
ESR1 | 0.53 | 4.47 |
ETS1 | 7.24 | 7.21 |
ELK1 | 10.74 | 9.15 |
TF, transcription factor; HCC, hepatocellular carcinoma.
Binding sites of ESR1 in lncRNA MEG3 promoter region
Figure 2A shows that the UCSC provided the binding motif of ESR1 and then predicted five potential binding sites for ESR1 in the lncRNA MEG3 promoter. In order to confirm the specific interaction between them, the luciferase reporter assay was performed (Figure 2B). ESR1 overexpression significantly increased the luciferase activity of lncRNA MEG3-WT promoter at site CCTGGCCGCCATGACCAA (P<0.01), but no alteration was observed in the sequence with mutated site X, which indicated that ESR1 bound to site AAGGTTCGGGTAAAGGTC in the lncRNA MEG3 promoter. Subsequently, the results of RIP assay also validated that the fragment of lncRNA MEG3 promoter region rather than its gene region was highly enriched in the anti-ESR1 groups (Figure 2C, P<0.001). These results verified the specific combination of ESR1 and lncRNA MEG3 promoter.

Effect of ESR1 on HepG2 cell proliferation
In order to probe the biological influence of ESR1 on HepG2 cells, ESR1 plasmid and empty vector, siRNAs targeting ESR1 and si-NC were transfected into HepG2 cells (Figure 3A,3B). The results of qRT-PCR showed that the ESR1 plasmid significantly increased ESR1 expression and the expression of ESR1 in cells transfected with siRNA-1014, 1380, and 1553 was lower than with NC-si, and the transfection efficiency of siRNA-1014 was best (P<0.05). Furthermore, the results of the CCK-8 test showed that overexpression of ESR1 suppressed cell proliferation as opposed to cells transfected with empty vector at HG, while knockdown of ESR1 promoted cell proliferation (Figure 3C,3D, P<0.05). The results of flow cytometry analysis displayed that overexpression of ESR1 promoted cell apoptosis compared to cells transfected with empty vector at HG, while knockdown of ESR1 repressed cell apoptosis (Figure 3E,3F, P<0.01).
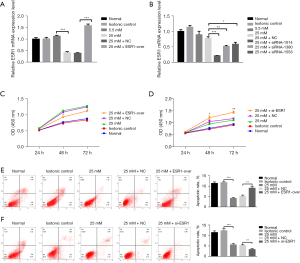
Effect of ESR1 on HepG2 cell migration and invasion
The outcome of the wound healing assay was that cells transfected with ESR1 plasmid displayed slower closing of scratch wounds compared to empty vector. Meanwhile, downregulation of ESR1 expression promoted cell migration (Figure 4A,4B, P<0.05). The Transwell assay provided evidence that overexpression of ESR1 significantly inhibited cell migration and invasiveness, whereas inhibition of ESR1 apparently enhanced HepG2 cell migration and invasion compared to the NC group (Figure 4C,4D, P<0.05). These results demonstrated that ESR1 might inhibit HepG2 cell proliferation, migration and invasion under HG conditions.
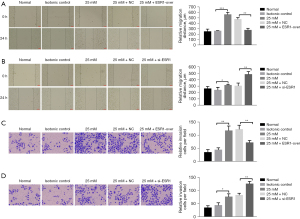
Effect of ESR1 on MEG3 regulation in HepG2 cells
In order to explore the effects of ESR1 on MEG3, the expression of MEG3 was detected in HepG2 cells with a plasmid expressing ESR1 and siRNA. The qRT-PCR results showed that overexpression of ESR1 increased MEG3 expression and underexpression of ESR1 decreased MEG3 expression when compared to the controls at HG (Figure 5A,5B, P<0.05). Rescue assays were performed to elucidate the positive regulatory effect of ESR1/MEG3 on the proliferation, migration, and invasion of HepG2 cells. The results of CCK-8 and colony formation assays showed that ESR1 overexpression suppressed HG-induced cell proliferation, but inhibition of MEG3 remarkably provoked cell proliferation (Figure 5C,5D, P<0.05). The results of flow cytometry analysis showed that ESR1 overexpression facilitated cell apoptosis at HG, but the promoting effect of ESR1 overexpression on cell apoptosis was reversed by MEG3 down-regulation (Figure 5E, P<0.05). The results of EdU assay displayed that ESR1 overexpression suppressed HG-induced cell proliferation, but the inhibitory effect of ESR1 overexpression on cell proliferation was reversed by MEG3 down-regulation (Figure 5F). Moreover, the wound healing and Transwell assays displayed that silencing MEG3 reversed the inhibitory effect of ESR1 overexpression on HepG2 cell migration and invasion (Figure 5G,5H, P<0.01).
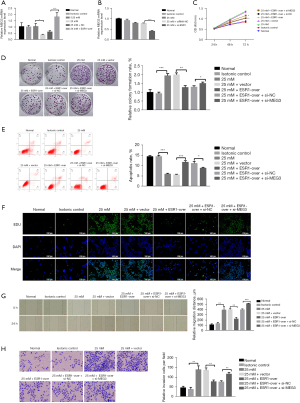
Discussion
DM is an independent risk factor for HCC because it can promote the occurrence and development of HCC through a variety of mechanisms, mainly hyperinsulinemia, hyperglycemia and chronic inflammation (8,9). However, the underlying mechanism of the association between HCC and DM is not fully understood and identifying a target to inhibit glucose-induced proliferation and migration would provide hope to HCC patients with DM. We investigated the underlying mechanism of ESR1 regulating lncRNA MEG3 in HG-treated HCC cells. We observed that HG promoted the proliferation, migration and invasion of HepG2 cells, but also downregulated ESR1 expression and ESR1 underexpression suppressed MEG3 expression and increased cell proliferation, migration and invasion. These results provided new insights into clinical treatment of DM-induced HCC and further intervention target.
LncRNAs are ncRNAs >200 nucleotides that play important roles in the regulation of tumor proliferation, migration and other physiological processes (28). MEG3, a type of lncRNA, plays a role in insulin production and secretion and the resistance of human islet cells (29-31). Emerging evidence suggests that MEG3 is associated with the development of tumors, and ectopic expression of MEG3 inhibits growth of tumor cells in cervical cancer, breast cancer and glioma (17). Several studies have demonstrated that MEG3 might predict the survival of HCC, and overexpression of MEG3 inhibits cell proliferation in HCC (32). In our previous research, MEG3 proved to be a bridge between DM and HCC. More specifically, the expression of MEG3 was decreased in HG-treated HepG2 cells, and overexpression of MEG3 inhibited proliferation and migration of HepG2 cells (15). Our findings strongly contributed to the development of lncRNA MEG3 as a promising prognostic biomarker and therapeutic strategy of DM-induced HCC.
TFs are an important factor affecting gene transcription in transcriptional regulation. Studies have shown that expression levels of TFs and promoter binding strength regulate the expression of lncRNAs. For example, the binding site of nuclear factor kappa B (NF-κB) is located in lncRNA AK019103 promoter and the expression level of lncRNA AK019103 is regulated by NF-κB (22). SOX2, POU5FL and Nanog regulate the expression of lncRNAs (33). So, we wondered whether HG regulates the role of MEG3 in the proliferation and migration of HepG2 cells via TFs. To confirm this notion, a bioinformatics analysis was performed to identify if ESR1 might act as a TF of MEG3. ESR1 is widely expressed in cells and plays a key role in both hormone-dependent and hormone-independent cancers. In hormone-related cancers, such as breast cancer and endometrial cancer, the expression of ESR1 mainly promotes development of the disease by regulating the PI3K/Akt pathway. ESR1 could act as an oncogene and promote development of prostate cancer by inducing the activation of epithelial-mesenchymal transition (EMT) and matrix metalloproteinases (34,35). However, ESR1 can also restore breast cancer sensitivity to hormone therapy (36). In our study, ESR1 was the upstream TF of MEG3 through prediction, screening and verification. The data revealed that ESR1 might be a tumor suppressor; however, HG suppressed expression of MEG3 to promote the proliferation and migration of HepG2 cells through downregulating the expression of ESR1. We speculate that the ESR1–MEG3 axis plays an important role in promoting the proliferation, migration and invasion of HepG2 cells under HG conditions. And the ESR1-MEG3 axis may have the potential to treat DM-induced HCC.
There were some limitations to this study. Firstly, we did not perform animal experiments to verify the results of this study. Secondly, although the results showed that ESR1 positively regulated lncRNA MEG3 in HG-treated HepG2 cells, the molecular signals or pathways involved in this process are still unknown. Thirdly, we failed to increase the research and analysis of miRNA data that may be more meaningful to construct a ceRNA network. Therefore, further investigations are needed to verify our in vitro findings and to explore in depth the underlying regulatory mechanism.
Last but not least, the application of lncRNAs into the clinic needs to overcome some problems and challenges, such as delivery, specificity and tolerability. Delivery problems are associated with the inefficient intracellular delivery, instability of ‘naked’ (chemically unmodified RNA structures) and lack of delivery vehicles suitable for targeting the cell type of interest. Specificity problems include off-target effects due to sequence similarity or overdosing to endogenous levels much higher than expected. Moreover, tolerability issues are associated with the recognition of RNA structures by pathogen-associated molecular pattern (PAMP) receptors.
Conclusions
ESR1 suppressed HG-induced proliferation, migration and invasion of HepG2 cells via positively regulating lncRNA MEG3. These findings present new ideas of a target for the treatment of HCC patients with DM.
Acknowledgments
Funding: This work was supported by the Tianjin Key Medical Discipline (Specialty) Construct Project (No. TJYXZDXK-032A); China International Medical Exchange Foundation Key Fund Project (No. Z-2017-26-1902, to Pei Yu); Whitehorn Diabetes Research Fund Project (No. G-X-2019-56, to Pei Yu); Tianjin Municipal Health Care Commission Scientific Research Fund Project (No. ZC20128, to Pei Yu); Tianjin Science and Technology Major Special Project and Engineering Public Health Science and Technology Major Special Project (No. 21ZXGWSY00100, to Pei Yu). The funders were not for profit.
Footnote
Reporting Checklist: The authors have completed the MDAR reporting checklist. Available at https://jgo.amegroups.com/article/view/10.21037/jgo-22-825/rc
Data Sharing Statement: Available at https://jgo.amegroups.com/article/view/10.21037/jgo-22-825/dss
Conflicts of Interest: All authors have completed the ICMJE uniform disclosure form (available at https://jgo.amegroups.com/article/view/10.21037/jgo-22-825/coif). PY reports that this work was supported by China International Medical Exchange Foundation Key Fund Project (No. Z-2017-26-1902, to PY); Whitehorn Diabetes Research Fund Project (No. G-X-2019-56, to PY); Tianjin Municipal Health Care Commission Scientific Research Fund Project (No. ZC20128, to PY); Tianjin Science and Technology Major Special Project and Engineering Public Health Science and Technology Major Special Project (No. 21ZXGWSY00100, to PY). The other authors have no conflicts of interest to declare.
Ethical Statement: The authors are accountable for all aspects of the work in ensuring that questions related to the accuracy or integrity of any part of the work are appropriately investigated and resolved. The study was conducted in accordance with the Declaration of Helsinki (as revised in 2013).
Open Access Statement: This is an Open Access article distributed in accordance with the Creative Commons Attribution-NonCommercial-NoDerivs 4.0 International License (CC BY-NC-ND 4.0), which permits the non-commercial replication and distribution of the article with the strict proviso that no changes or edits are made and the original work is properly cited (including links to both the formal publication through the relevant DOI and the license). See: https://creativecommons.org/licenses/by-nc-nd/4.0/.
References
- Forner A, Reig M, Bruix J. Hepatocellular carcinoma. Lancet 2018;391:1301-14. [Crossref] [PubMed]
- Kaka B, Maharaj SS. Effect of Rebound Exercises and Circuit Training on Complications Associated with Type 2 Diabetes: Protocol for a Randomized Controlled Trial. JMIR Res Protoc 2018;7:e124. [Crossref] [PubMed]
- El-Serag HB, Hampel H, Javadi F. The association between diabetes and hepatocellular carcinoma: a systematic review of epidemiologic evidence. Clin Gastroenterol Hepatol 2006;4:369-80. [Crossref] [PubMed]
- Butt AA, Khan UA, McGinnis KA, et al. Co-morbid medical and psychiatric illness and substance abuse in HCV-infected and uninfected veterans. J Viral Hepat 2007;14:890-6. [Crossref] [PubMed]
- Sudharsanan N, Ali MK, Mehta NK, et al. Population aging, macroeconomic changes, and global diabetes prevalence, 1990-2008. Popul Health Metr 2015;13:33. [Crossref] [PubMed]
- Tanaka K, Tsuji I, Tamakoshi A, et al. Diabetes mellitus and liver cancer risk: an evaluation based on a systematic review of epidemiologic evidence among the Japanese population. Jpn J Clin Oncol 2014;44:986-99. [Crossref] [PubMed]
- Rousseau MC, Parent ME, Pollak MN, et al. Diabetes mellitus and cancer risk in a population-based case-control study among men from Montreal, Canada. Int J Cancer 2006;118:2105-9. [Crossref] [PubMed]
- Polesel J, Zucchetto A, Montella M, et al. The impact of obesity and diabetes mellitus on the risk of hepatocellular carcinoma. Ann Oncol 2009;20:353-7. [Crossref] [PubMed]
- Chen CT, Chen JY, Wang JH, et al. Diabetes mellitus, metabolic syndrome and obesity are not significant risk factors for hepatocellular carcinoma in an HBV- and HCV-endemic area of Southern Taiwan. Kaohsiung J Med Sci 2013;29:451-9. [Crossref] [PubMed]
- Fong DG, Nehra V, Lindor KD, et al. Metabolic and nutritional considerations in nonalcoholic fatty liver. Hepatology 2000;32:3-10. [Crossref] [PubMed]
- Angulo P, Keach JC, Batts KP, et al. Independent predictors of liver fibrosis in patients with nonalcoholic steatohepatitis. Hepatology 1999;30:1356-62. [Crossref] [PubMed]
- Ford ES, Cogswell ME. Diabetes and serum ferritin concentration among U.S. adults. Diabetes Care 1999;22:1978-83. [Crossref] [PubMed]
- Fain JN. Release of inflammatory mediators by human adipose tissue is enhanced in obesity and primarily by the nonfat cells: a review. Mediators Inflamm 2010;2010:513948. [Crossref] [PubMed]
- Goyal R, Faizy AF, Siddiqui SS, et al. Evaluation of TNF-α and IL-6 Levels in Obese and Non-obese Diabetics: Pre- and Postinsulin Effects. N Am J Med Sci 2012;4:180-4. [Crossref] [PubMed]
- Li X, Cheng T, He Y, et al. High glucose regulates ERp29 in hepatocellular carcinoma by LncRNA MEG3-miRNA 483-3p pathway. Life Sci 2019;232:116602. [Crossref] [PubMed]
- He Y, Luo Y, Liang B, et al. Potential applications of MEG3 in cancer diagnosis and prognosis. Oncotarget 2017;8:73282-95. [Crossref] [PubMed]
- Zhang X, Zhou Y, Mehta KR, et al. A pituitary-derived MEG3 isoform functions as a growth suppressor in tumor cells. J Clin Endocrinol Metab 2003;88:5119-26. [Crossref] [PubMed]
- Nathan DM, Buse JB, Davidson MB, et al. Medical management of hyperglycaemia in type 2 diabetes mellitus: a consensus algorithm for the initiation and adjustment of therapy: a consensus statement from the American Diabetes Association and the European Association for the Study of Diabetes. Diabetologia 2009;52:17-30. [Crossref] [PubMed]
- Evans JM, Donnelly LA, Emslie-Smith AM, et al. Metformin and reduced risk of cancer in diabetic patients. BMJ 2005;330:1304-5. [Crossref] [PubMed]
- Donadon V, Balbi M, Ghersetti M, et al. Antidiabetic therapy and increased risk of hepatocellular carcinoma in chronic liver disease. World J Gastroenterol 2009;15:2506-11. [Crossref] [PubMed]
- Donadon V, Balbi M, Valent F, et al. Glycated hemoglobin and antidiabetic strategies as risk factors for hepatocellular carcinoma. World J Gastroenterol 2010;16:3025-32. [Crossref] [PubMed]
- Wan G, Hu X, Liu Y, et al. A novel non-coding RNA lncRNA-JADE connects DNA damage signalling to histone H4 acetylation. EMBO J 2013;32:2833-47. [Crossref] [PubMed]
- Theodoropoulou S, Papadopoulou A, Karapanou O, et al. Study of Xbal and Pvull polymorphisms of estrogen receptor alpha (ERα) gene in girls with precocious/early puberty. Endocrine 2021;73:455-62. [Crossref] [PubMed]
- Zhou G, Liu L, Li X, et al. ESRα Promoter Methylation May Modify the Association Between Lipid Metabolism and Type 2 Diabetes in Chinese Farmers. Front Public Health 2021;9:578134. [Crossref] [PubMed]
- Xu LW, Gou X, Yang JY, et al. Methylation of ERβ 5'-untranslated region attenuates its inhibitory effect on ERα gene transcription and promotes the initiation and progression of papillary thyroid cancer. FASEB J 2021;35:e21516. [Crossref] [PubMed]
- Guo S, Zhang B, Qi W, et al. Role of estrogen receptor alpha in MEHP-induced proliferation and invasion of SH-SY5Y cells. Toxicology 2021;453:152734. [Crossref] [PubMed]
- Wang C, Zeng H, Zhang L, et al. Sensitive quantitation of ESR1 mutations in cell-free DNA from breast cancer patients using base-specific invasive reaction assisted qPCR. J Pharm Biomed Anal 2021;197:113959. [Crossref] [PubMed]
- Prensner JR, Chinnaiyan AM. The emergence of lncRNAs in cancer biology. Cancer Discov 2011;1:391-407. [Crossref] [PubMed]
- You L, Wang N, Yin D, et al. Downregulation of Long Noncoding RNA Meg3 Affects Insulin Synthesis and Secretion in Mouse Pancreatic Beta Cells. J Cell Physiol 2016;231:852-62. [Crossref] [PubMed]
- Kameswaran V, Bramswig NC, McKenna LB, et al. Epigenetic regulation of the DLK1-MEG3 microRNA cluster in human type 2 diabetic islets. Cell Metab 2014;19:135-45. [Crossref] [PubMed]
- Wang N, Zhu Y, Xie M, et al. Long Noncoding RNA Meg3 Regulates Mafa Expression in Mouse Beta Cells by Inactivating Rad21, Smc3 or Sin3α. Cell Physiol Biochem 2018;45:2031-43. [Crossref] [PubMed]
- Zhuo H, Tang J, Lin Z, et al. The aberrant expression of MEG3 regulated by UHRF1 predicts the prognosis of hepatocellular carcinoma. Mol Carcinog 2016;55:209-19. [Crossref] [PubMed]
- Dinger ME, Amaral PP, Mercer TR, et al. Long noncoding RNAs in mouse embryonic stem cell pluripotency and differentiation. Genome Res 2008;18:1433-45. [Crossref] [PubMed]
- Tian W, Teng F, Gao J, et al. Estrogen and insulin synergistically promote endometrial cancer progression via crosstalk between their receptor signaling pathways. Cancer Biol Med 2019;16:55-70. [Crossref] [PubMed]
- Arun A, Ansari MI, Popli P, et al. New piperidine derivative DTPEP acts as dual-acting anti-breast cancer agent by targeting ERα and downregulating PI3K/Akt-PKCα leading to caspase-dependent apoptosis. Cell Prolif 2018;51:e12501. [Crossref] [PubMed]
- Mishra S, Tai Q, Gu X, et al. Estrogen and estrogen receptor alpha promotes malignancy and osteoblastic tumorigenesis in prostate cancer. Oncotarget 2015;6:44388-402. [Crossref] [PubMed]