Human chorionic gonadotropin-β promotes pancreatic cancer progression via the epithelial mesenchymal transition signaling pathway
Introduction
Pancreatic cancer is one of the most common causes of cancer-related deaths in the world, with 458,918 new pancreatic cancer patients in 2018, causing 432,242 deaths (1). Pancreatic cancer is often detected at an advanced stage and has a poor prognosis despite new chemotherapy and improved surgical techniques (2). In advanced pancreatic cancer, the 5-year survival rate is less than 5% even with chemotherapy (3). A better understanding of the molecular mechanisms involved in tumor progression and the migration of pancreatic cancer is crucial for the development of targeted treatments to improve the prognosis of patients with pancreatic cancer.
Human chorionic gonadotropin (hCG) is a heterodimeric placental glycoprotein hormone with α and β subunits, of which hCGβ is structurally homologous to the cysteine knot family of growth factors composed of transforming growth factor (TGF), platelet-derived growth factor (PDGF), and nerve growth factor (NGF) (4). Although hCGβ stabilizes the production of progesterone, which is important for pregnancy, it is also highly expressed in various cancers (5). Although hCGβ is often elevated in germ cell tumors such as placental-derived tumors and testicular tumors, hCGβ can also be overexpressed in undifferentiated gastrointestinal cancers, and its expression has been reported as a possible factor associated with poor prognoses (6,7). As mentioned earlier, hCGβ is structurally homologous to TGFβ, which is strongly involved in epithelial to mesenchymal transition (EMT), suggesting that they could have similar receptor-mediated effects in cancer cells (8). Although hCGβ is involved in cancer metastasis and invasion via EMT in colorectal and ovarian cancers (9,10), the molecular mechanisms remain unclear. In pancreatic cancer, hCGβ is also ectopically expressed (11,12). However, its association with prognoses and its molecular role have not been elucidated. We speculated that hCGβ may be a poor prognostic factor in pancreatic, colon and breast cancers. Elucidating the molecular role of hCGβ in pancreatic cancer progression may lead to hCGβ becoming a new molecular target for pancreatic cancer therapy.
In the present study, we analyzed the correlation between hCGβ expression and clinicopathological features in pancreatic cancer. We also investigated the molecular roles of hCGβ expression in pancreatic cancer cell lines, especially in the proliferative, migratory, and invasive abilities of cells. We present the following article in accordance with the MDAR reporting checklist (available at https://jgo.amegroups.com/article/view/10.21037/jgo-21-907/rc).
Methods
Patients
In the present study, we included 35 patients with pancreatic ductal carcinoma who underwent pancreatectomy at the University of the Ryukyus Hospital (Okinawa, Japan) between January 2011 and May 2020. We excluded 5 patients because of the presence of cancer in other organs, leaving a final cohort of 30 patients. We followed up the patients at 1- to 6-month intervals until death or September 30, 2020, whichever occurred earlier. This study was conducted in accordance with the Declaration of Helsinki (as revised in 2013). This study was approved by the Internal Review Board on Ethical Issues of the University of the Ryukyus (Approval No. 1600) and informed consent was obtained from all patients. Tumor staging was classified according to the Tumor Node Metastasis Classification of Malignant Tumors defined by the Union for International Cancer Control (Eighth edition).
hCGβ immunostaining of surgical specimens
We obtained formalin-fixed, paraffin-embedded blocks from the 30 patients and selected the blocks containing the cancerous areas. We obtained a total of 30 cancerous lesions, 1 each from 30 patients. We performed hCGβ immunohistochemistry on the 30 selected blocks. Briefly, after deparaffinization and rehydration, we incubated the tissue sections with a rabbit polyclonal antibody against hCGβ (1:350; A0231; Dako, Tokyo, Japan), followed by reaction with a dextran polymer reagent combined with secondary antibodies and peroxidase (Envision/HRP; Dako). We performed the assessment of hCGβ expression using an immunoreactive score (IRS) for each cancerous lesion, calculated by multiplying the staining range (%) by the intensity (0, negative; 1, weak; 2, moderate; 3, strong) of the stained cells. We defined cancer lesions with detected hCGβ staining at ×40, ×100, and ×200 magnification as strong, moderate, and weak, respectively. Two independent pathologists (K.T. and H.K) who were blinded to clinical outcomes performed the hCGβ staining evaluation. We defined cases with an intensity ≥1 as hCGβ expression-positive, divided the 30 cases into 2 groups, positive and negative, and compared their clinicopathological characteristics.
Cell culture
We employed two human pancreatic cancer cell lines, culturing AsPC1 and PK45H cell lines in Roswell Park Memorial Institute-1640 medium with 10% fetal bovine serum (FBS). The cell lines were maintained at 37 ℃ under 5% CO2.
hCGβ knockdown with small hairpin RNA
We transfected the AsPC1 and PK45H cells with hCGβ small hairpin RNA (shRNA) (MISSION® shRNA Library, TRCN0000082825, Sigma-Aldrich, St. Louis, MO, USA) using X-tremeGENETM HP DNA Transfection Reagent (Sigma-Aldrich) according to the manufacturer’s instructions. As a negative control (scramble), we employed MISSION pLKO.1-puro-Non-Mammalian shRNA Control (SHC002, Sigma-Aldrich). We harvested the cells at 48 hours post-transfection for future experiments. Successful downregulation of hCGβ expression in the ASPC1 and PK45H cells was verified by western blotting.
Western blotting
We lysed the samples with radioimmunoprecipitation assay lysis buffer, centrifuged them for 1 minute at 10,000×g, and performed a Bradford protein assay to measure the amounts of protein. After we added sodium dodecyl sulfate-polyacrylamide gel electrophoresis (SDS-PAGE) loading buffer (4% SDS, 20% glycerol, 10% β-mercaptoethanol, bromphenol blue, and 0.125 M Tris) to cytoplasmic protein obtained from the cell lines, the mixtures were incubated at 95 ℃ for 3 minutes. We performed the SDS-PAGE using 12% acrylamide gel, transferring the proteins (15 µg) onto polyvinylidene difluoride membrane with the Trans-Blot® TurboTM Transfer Kit (BIO-RAD, Hercules, CA, USA). We blocked the membrane proteins in 5% bovine serum albumin with Tris-buffered saline and Tween-20 for 20 minutes at room temperature. We incubated the membranes with primary antibody at 4 ℃ overnight, and then incubated them with m-IgGk BP-HRP (1:10000; sc-516102; Santa Cruz Biotechnology, Dallas, TX, USA) as a secondary antibody. We employed the following primary antibodies: hCGβ (1:500; sc-271062), E-cadherin (1:500, sc-8426), vimentin (1:500, sc-6260), slug (1:500, sc-166476), and α-smooth muscle actin (SMA) (1:500, sc-53142), (all Santa Cruz Biotechnology) and β-actin (1:500, #12620, Cell Signaling Technology). After we washed the membranes with phosphate-buffered saline and Tween-20 3 times, we added peroxidase luminescent matrix to the membrane. We visualized the membrane using a luminescent image analyzer (LAS4000; Cytiva, Marlborough, MA, USA).
Cell proliferation assay
We measured the cell proliferation capacity employing the Premix WST-1 Cell Proliferation Assay System (TAKARA BIO INC, Otsu, JAPAN). We incubated the AsPC1 and PK45H scramble and knockdown cell lines for 24, 48, and 96 hours at 37 ℃ under 5% CO2, then added 10 µL of Premix WST-1 and incubated them again at 37 ℃ under 5% CO2 for 1 hour. We measured the absorbance of the formazan products using a microplate reader (SH-1000 Lab, Corona Electric Co, Hitachi, Japan). We performed all tests 3 times each.
Invasion assay
The cultured AsPC1 and PK45H scramble and knockdown cell lines were trypsinized and centrifuged to remove dead cells and cell debris. We then added serum-free medium and added a total of 500 µL to each Corning Matrigel Invasion Chamber 24-Well Plate 8.0 micron culture insert (Corning Life Sciences, Durham, NC, USA). We placed the inserts in individual wells prepared with 750 µL of medium with 10% FBS and incubated them for 24 hours at 37 ℃ under 5% CO2. After removing the inserts from the wells and wiping off the medium, we wiped the inside of the inserts with a cotton swab. This operation was performed carefully to avoid touching the outside of the inserts. We stained the cells attached to the outside of the insert using Diff-Quik stainTM (Sysmex Corporation, Kobe, Japan), and their numbers were measured by two blinded researchers using a light microscope. The number of invaded cells was taken as the average of the numbers measured in multiple fields of view, and we calculated the invasion rate (%) (number of invaded cells in the sample/number of invaded cells in the control × 100. We performed all tests 3 times each.
Wound healing assay
We trypsinized and centrifuged cultured AsPC1 and PK45H scramble and knockdown cell lines to remove dead cells and cell debris, then added serum-free medium and applied a total of 70 µL to each culture dish containing ibidi culture inserts (ibidi, Martinsried, Germany). After incubation for 24 hours at 37 ℃ under 5% CO2, we removed the culture inserts using sterile forceps to prevent the cell layer from peeling off. We then washed the wells with phosphate-buffered saline and added 2 mL of serum-free medium to prevent cell proliferation. We visualized cell migration to 500 µm, the width of the cell-free gap, under a light microscope and measured the closure rate (%) (migrated cell surface area/total surface area ×100) after 24 and 48 hours. We performed all tests 3 times each.
Statistical analysis
We employed chi-squared tests for comparisons of categorical variables (such as sex) and Mann-Whitney U tests for comparisons of continuous variables (such as age) between the hCGβ expression-positive and hCGβ expression-negative groups. We estimated survival using Kaplan-Meier curves. Correlations between clinical outcome and hCGβ expression were assessed with the log-rank test. We calculated overall survival (OS) from the date of surgery to the date of death from any cause. Recurrence- free survival (RFS) was calculated from the date of surgery to the date of disease recurrence or death from any cause. We used the Cox hazard regression method to identify independent risk factors for OS and RFS. For all the statistical analyses, we considered 2-tailed P values of 0.05 or less as statistically significant. We performed all statistical analyses with IBM SPSS Statistics version 24 (IBM, Armonk, NY, USA).
Results
Clinicopathological features of cases with hCGβ expression in pancreatic cancer tissue of 30 patients who underwent hCGβ immunostaining in pancreatic cancer tissue were positive in 21 (70%). Some of the immunostaining results of the primary lesions are shown in Figure 1A. With respect to age, sex, primary site, stage, histopathology, and with or without adjuvant chemotherapy, there were no significant differences between the hCGβ-positive and -negative groups (Table 1). However, the presence of recurrence (P=0.011), CEA levels (P=0.001), and CA19-9 levels (P=0.043) were significantly associated with hCGβ expression (Table 1).
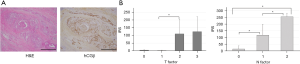
Table 1
Clinical characteristics | Total (N=30) | hCGβ-negative (N=9) | hCGβ-positive (N=21) | P value |
---|---|---|---|---|
Age | 0.673 | |||
Median | 63 | 63 | 63 | |
Sex | 0.376 | |||
Male | 17 | 4 | 13 | |
Female | 13 | 5 | 8 | |
Pancreatic location of the tumor | 0.193 | |||
Head | 12 | 2 | 10 | |
Body and tail | 18 | 7 | 11 | |
pT factor | 0.108 | |||
pTis | 4 | 2 | 2 | |
pT1 | 4 | 3 | 1 | |
pT2 | 11 | 2 | 9 | |
pT3 | 11 | 2 | 9 | |
pT4 | 0 | 0 | 0 | |
pN factor | 0.418 | |||
Negative | 13 | 5 | 8 | |
pN1 | 14 | 4 | 10 | |
pN2 | 3 | 0 | 3 | |
pStage | 0.730 | |||
pPanIN1-3 | 4 | 2 | 2 | |
pStage IA | 2 | 1 | 1 | |
pStage IB | 4 | 1 | 3 | |
pStage IIA | 3 | 1 | 2 | |
pStage IIB | 14 | 4 | 10 | |
pStage III | 3 | 0 | 3 | |
pStage IV | 0 | 0 | 0 | |
Histopathology | 0.388 | |||
Well | 4 | 2 | 2 | |
Moderately | 13 | 2 | 11 | |
Poor | 4 | 1 | 3 | |
Others | 9 | 4 | 5 | |
Adjuvant chemotherapy | 0.936 | |||
Yes | 17 | 5 | 12 | |
No | 13 | 4 | 9 | |
Recurrence | 0.011 | |||
Yes | 20 | 3 | 17 | |
No | 10 | 6 | 4 | |
Tumor marker | ||||
CEA median | 3 | 2 | 3.5 | 0.001 |
CA19-9 median | 62 | 28 | 257.5 | 0.043 |
There were no statistically significant differences between the two groups. hCGβ, human chorionic gonadotropin free beta-subunit; CEA, carcinoembryonic antigen; CA19-9, carbohydrate antigen 19-9.
Association between IRS and tumor progression
To clarify the association between hCGβ expression and tumor progression, we performed an association analysis of the IRS with T and N factors. There was no significant increase in the IRS between T0 and T1, but there was a significant increase between T1 and T2, and no significant increase between T2 and T3 (Figure 1B). For the N factor, the IRS was significantly elevated from N0 to N1 and from N1 to N2 (Figure 1B).
Association between hCGβ expression in pancreatic cancer tissue and prognosis
We next performed a Kaplan-Meier analysis to assess the prognostic value of hCGβ expression in pancreatic cancer. Using the log-rank test, we found a significant association between hCGβ expression (vs. nonexpression) in pancreatic cancer and both OS and RFS (19 vs. 77 months for OS and 11 vs. 74 months for RFS for hCGβ expression and nonexpression, respectively, both P<0.001; Figure 2). Moreover, we performed univariate and multivariate Cox proportional hazard regression analyses, summarized in Table 2. In the univariate analysis, hCGβ expression was significantly associated with OS [hazard ratio (HR) 23.5; 95% CI: 2.9–190 months; P=0.003] and RFS (HR 6.0; 95% CI: 1.3–27 months; P=0.02). In the multivariate analysis, hCGβ expression was the only independent factor for OS (HR 14; 95% CI: 1.5–130 months; P=0.0019). Although not significant, hCGβ-positive patients tended to have poorer RFS in the multivariate analysis (HR 4.1; 95% CI: 0.87–19 months; P=0.074).
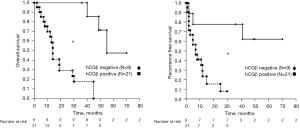
Table 2
Univariate analysis | Multivariate analysis | ||||||||
---|---|---|---|---|---|---|---|---|---|
HR | Lower 95% CI | Upper 95% CI | P value | HR | Lower 95% CI | Upper 95% CI | P value | ||
Overall survival | |||||||||
Age (<65 or ≥65 years) | 0.76 | 0.30 | 1.9 | 0.57 | |||||
Sex (male or female) | 0.54 | 0.21 | 1.4 | 0.20 | |||||
hCGβ (negative or positive) | 23.5 | 2.9 | 190 | 0.003 | 14 | 1.5 | 130 | 0.019 | |
CA19-9 (<37 U/mL or ≥37 U/mL) | 2.4 | 0.80 | 6.9 | 0.12 | 1.1 | 0.32 | 3.7 | 0.9 | |
CEA (<5 ng/mL or ≥5 ng/mL) | 3.8 | 1.34 | 10.8 | 0.010 | 1.9 | 0.60 | 6.0 | 0.28 | |
Stage (I or ≥II) | 5.9 | 0.79 | 44.6 | 0.083 | 3.6 | 0.41 | 32 | 0.25 | |
T factor (1, 2 or 3) | 3.1 | 1.25 | 7.8 | 0.015 | 1.6 | 0.58 | 4.5 | 0.36 | |
N factor (0 or 1, 2) | 1.9 | 0.72 | 5.1 | 0.19 | |||||
Recurrence free survival | |||||||||
Age (<65 or ≥65 years) | 0.55 | 0.21 | 1.4 | 0.21 | |||||
Sex (male or female) | 0.58 | 0.23 | 1.5 | 0.25 | |||||
hCGβ (negative or positive) | 6.0 | 1.3 | 27 | 0.02 | 4.1 | 0.87 | 19 | 0.074 | |
CA19-9 (<37 U/mL or ≥37 U/mL) | 1.5 | 0.56 | 4.1 | 0.41 | |||||
CEA (<5 ng/mL or ≥5 ng/mL) | 2.5 | 0.95 | 6.8 | 0.064 | 1.1 | 0.36 | 3.1 | 0.91 | |
Stage (I or ≥II) | 6.9 | 0.92 | 52 | 0.060 | 3.4 | 0.41 | 28 | 0.25 | |
T factor (1, 2 or 3) | 3.9 | 1.5 | 1.8 | 0.004 | 2.4 | 0.87 | 6.6 | 0.090 | |
N factor (0 or 1, 2) | 2.3 | 0.87 | 6.2 | 0.092 |
hCGβ positivity was an independent associated factor in overall survival. Although hCGβ positivity was not a statistically significant associated factor in recurrence free survival, it showed a very strong association. hCGβ, human chorionic gonadotropin free beta-subunit; CEA, carcinoembryonic antigen; CA19-9, carbohydrate antigen 19-9.
Association between hCGβ expression and EMT
To examine the potential molecular mechanisms of hCGβ in EMT, we performed western blotting to detect the expression of epithelial and mesenchymal protein markers. The results showed that the hCGβ-downregulated AsPC1 and PK45H cells demonstrated increased E-cadherin expression and reduced vimentin, slug, and α-SMA expression (Figure 3).
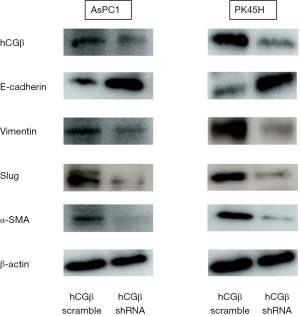
Cell proliferative capacity
We performed a WST-1 assay at 24, 48, and 72 hours after seeding the cell lines. The results of the WST-1 assay are shown in Figure 4. In both AsPC1 and PK45H, the cell proliferation of the hCGβ shRNA-transfected cell lines was significantly reduced (P<0.05).
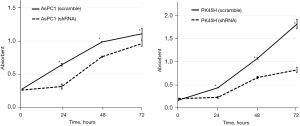
Invasion capacity
In both AsPC1 and PK45H, the invasion of the hCGβ shRNA-transfected cells was significantly inhibited compared with the negative control (P<0.05) (Figure 5A).
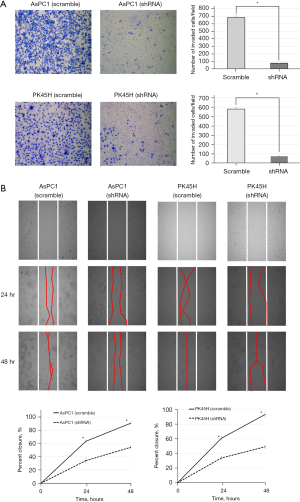
Migratory ability
Intercellular spaces were measured at 24 and 48 hours after cell seeding. In both AsPC1 and PK45H, the closure rate of the hCGβ shRNA-transfected cell lines was significantly reduced compared with the control (Figure 5B).
Discussion
To our knowledge, this study is the first to examine the relationship between hCGβ expression and tumor progression in pancreatic cancer. We investigated the clinicopathological significance of hCGβ expression in pancreatic cancer and the molecular role of hCGβ in pancreatic cancer progression. The results of immunostaining revealed that hCGβ-positive patients had a significantly higher recurrence rate, higher tumor markers, and a poorer prognosis. These results suggest that hCGβ is involved in tumor growth in pancreatic cancer. In addition, the IRS of hCGβ correlated with tumor T and N factors. These results also support the above-stated theory. Ectopic expression of hCGβ has been found in many epithelial carcinomas, including pancreatic cancer, and all of them had poor prognostic factors (13-15). Given the results of the present study were in agreement with the above finding, hCGβ could be a novel prognostic marker in pancreatic cancer.
Next, 2 hCGβ-downregulated pancreatic cancer cell lines were used to confirm the molecular effects of hCGβ in prompting cell proliferation, migration, and invasion. The hCGβ-downregulated cell lines had significantly reduced proliferative ability, invasion capacity, and migratory ability compared with the control cell lines. Moreover, the western blot analysis showed that the expression of cadherin, an epithelial marker, was upregulated, and the expressions of the mesenchymal markers vimentin, slug, and α-SMA were downregulated in the hCGβ shRNA-transfected pancreatic cancer cell lines compared with the control cell lines. Downregulation of epithelial markers and upregulation of mesenchymal markers occur during EMT, which is one of the key processes by which cancers acquire invasive and metastatic properties (16,17). Therefore, the results of the present study suggest that hCGβ induces EMT in pancreatic cancer cells, resulting in the cells acquiring proliferative, invasive, and migratory capacities.
The biological role of tumor-produced hCGβ in cancer is not completely understood. Most choriocarcinomas and germ cell tumors produce an intact heterodimeric hormone composed of alpha and beta subunits; however, many nonchorionic tumors produce only hCGβ (18). hCGβ is structurally homologous to the cysteine knot family of growth factors consisting of TGF, PDGF, and NGF, and also has a 4-peptide cysteine knot structure in the β-subunit in common with TGFβ (4,8). In particular, it is important that hCGβ has structural homology with TGFβ, which is a significant inducer of EMT. In early-stage pancreatic cancer, TGFβ plays a role as a tumor suppressor by promoting apoptosis and inhibiting epithelial cell cycle progression; in its advanced stage, however, TGFβ plays a role as a tumor progression factor based on genomic instability, neoangiogenesis, immune evasion, cell motility, and metastasis (19). In addition, TGFβ might induce apoptosis and tumor suppression in cancer cells, induce EMT, or promote cancer stem cell heterogeneity and drug resistance (20,21). The structural homology of hCGβ to TGFβ suggests that tumor-produced hCGβ might bind to TGF β receptor complex components, blocking their ligand binding sites, thus inhibiting their interaction with other receptor components necessary to initiate the cytoplasmic signaling and leading to apoptosis (7). Furthermore, hyperglycosylated hCGβ shows a potent angiogenic effect through the activation of TGFβ (22). Ectopically expressed hCGβ not only has an anti-apoptotic effect, but also promotes invasion capacity by downregulating E-cadherin, which plays an important role in epithelial cell-to-cell adhesion and is an invasion suppressor (6,23). Further clarification of the hCGβ-related pathways underlying the interaction between hCGβ and TGFβ receptors will lead to the development of targeted therapeutics such as hCGβ antagonists, which are expected to be used in pancreatic cancer treatment. To elucidate the hCGβ-related pathway underlying the interaction between hCGβ and TGFβ receptors, it is necessary to confirm not only hCGβ expression but also TGFβ and TGFβ receptor expression in pancreatic cancer cells; therefore, further research is warranted. Although speculative, other hCGβ-activated receptors, such as the hCG/LH receptor, may be important in pancreatic cancer progression, and further studies are also needed.
In the future, hCGβ measurements could change the progression diagnosis and treatment of pancreatic cancer. The addition of hCGβ immunostaining to pathology could allow for a more detailed determination of pancreatic cancer progression. Stronger adjuvant chemotherapy could be required for patients with positive hCGβ immunostaining, even if the histopathology shows early-stage cancer. Although not investigated in the present study, measurement of serum hCGβ levels could also be useful as a prognostic factor. It has been reported that patients with high serum hCGβ levels had poor prognoses in non-germ cell carcinoma, including colorectal and gastric cancer (13,24-27), and similar results might be obtained in pancreatic cancer. It could also be useful to determine the effects of treatment by performing serial measurements during chemotherapy.
Several limitations of this study should be acknowledged. First, the number of cases for hCGβ immunostaining was small and there was a slight bias in the number of cases between the hCGβ-positive and hCGβ-negative groups. With a larger number of cases, a multivariate analysis might have revealed the statistical relevance of hCGβ expression in RFS. Future studies with large sample sizes are required to accurately evaluate hCGβ. Second, we did not conduct comparative experiments using hCGβ-upregulated pancreatic cancer cell lines. We used only hCGβ-downregulated cell lines and were able to show a reduction in cell proliferation, invasion, and migration; however, it might have been desirable to show the opposite results using hCGβ-upregulated cell lines.
In summary, this present study suggests that hCGβ promotes the EMT signaling pathway, which in turn stimulates pancreatic cancer invasion and metastasis. In the future, novel agents targeting hCGβ are expected to improve the prognosis of patients with pancreatic cancer.
Acknowledgments
The authors would like to thank Ms. Matsushita for technical assistance with the experiments. We also thank Ms. Nakaza for technical assistance. We thank all other members and staff for their contributions to sample collection and the completion of our study.
Funding: None.
Footnote
Reporting Checklist: The authors have completed the MDAR reporting checklist. Available at https://jgo.amegroups.com/article/view/10.21037/jgo-21-907/rc
Data Sharing Statement: Available at https://jgo.amegroups.com/article/view/10.21037/jgo-21-907/dss
Conflicts of Interest: All authors have completed the ICMJE uniform disclosure form (available at https://jgo.amegroups.com/article/view/10.21037/jgo-21-907/coif). The authors have no conflicts of interest to declare.
Ethical Statement: The authors are accountable for all aspects of the work in ensuring that questions related to the accuracy or integrity of any part of the work are appropriately investigated and resolved. This study was conducted in accordance with the Declaration of Helsinki (as revised in 2013). This study was approved by the Internal Review Board on Ethical Issues of the University of the Ryukyus (Approval No. 1600) and informed consent was obtained from all patients.
Open Access Statement: This is an Open Access article distributed in accordance with the Creative Commons Attribution-NonCommercial-NoDerivs 4.0 International License (CC BY-NC-ND 4.0), which permits the non-commercial replication and distribution of the article with the strict proviso that no changes or edits are made and the original work is properly cited (including links to both the formal publication through the relevant DOI and the license). See: https://creativecommons.org/licenses/by-nc-nd/4.0/.
References
- Rawla P, Sunkara T, Gaduputi V. Epidemiology of Pancreatic Cancer: Global Trends, Etiology and Risk Factors. World J Oncol 2019;10:10-27. [Crossref] [PubMed]
- Kamisawa T, Wood LD, Itoi T, et al. Pancreatic cancer. Lancet 2016;388:73-85. [Crossref] [PubMed]
- Vivaldi C, Fornaro L, Vasile E. FOLFIRINOX Adjuvant Therapy for Pancreatic Cancer. N Engl J Med 2019;380:1187-8. [Crossref] [PubMed]
- Hearn MT, Gomme PT. Molecular architecture and biorecognition processes of the cystine knot protein superfamily: part I. The glycoprotein hormones. J Mol Recognit 2000;13:223-78. [Crossref] [PubMed]
- Huhtaniemi I, Rulli S, Ahtiainen P, et al. Multiple sites of tumorigenesis in transgenic mice overproducing hCG. Mol Cell Endocrinol 2005;234:117-26. [Crossref] [PubMed]
- Iles RK, Delves PJ, Butler SA. Does hCG or hCGβ play a role in cancer cell biology?. Mol Cell Endocrinol 2010;329:62-70. [Crossref] [PubMed]
- Iles RK. Ectopic hCGbeta expression by epithelial cancer: malignant behaviour, metastasis and inhibition of tumor cell apoptosis. Mol Cell Endocrinol 2007;260-262:264-270. [Crossref] [PubMed]
- Hiro'oka T, Maassen D, Berger P, et al. Disulfide bond mutations in follicle-stimulating hormone result in uncoupling of biological activity from intracellular behavior. Endocrinology 2000;141:4751-6. [Crossref] [PubMed]
- Kawamata F, Nishihara H, Homma S, et al. Chorionic Gonadotropin-β Modulates Epithelial-Mesenchymal Transition in Colorectal Carcinoma Metastasis. Am J Pathol 2018;188:204-15. [Crossref] [PubMed]
- Liu N, Peng SM, Zhan GX, et al. Human chorionic gonadotropin β regulates epithelial-mesenchymal transition and metastasis in human ovarian cancer. Oncol Rep 2017;38:1464-72. [Crossref] [PubMed]
- Louhimo J, Nordling S, Alfthan H, et al. Specific staining of human chorionic gonadotropin beta in benign and malignant gastrointestinal tissues with monoclonal antibodies. Histopathology 2001;38:418-24. [Crossref] [PubMed]
- Syrigos KN, Fyssas I, Konstandoulakis MM, et al. Beta human chorionic gonadotropin concentrations in serum of patients with pancreatic adenocarcinoma. Gut 1998;42:88-91. [Crossref] [PubMed]
- Louhimo J, Kokkola A, Alfthan H, et al. Preoperative hCGbeta and CA 72-4 are prognostic factors in gastric cancer. Int J Cancer 2004;111:929-33. [Crossref] [PubMed]
- Butler SA, Ikram MS, Mathieu S, et al. The increase in bladder carcinoma cell population induced by the free beta subunit of human chorionic gonadotrophin is a result of an anti-apoptosis effect and not cell proliferation. Br J Cancer 2000;82:1553-6. [PubMed]
- Konishi Y, Kawamata F, Nishihara H, et al. Tumor budding and human chorionic gonadotropin-β expression correlate with unfavorable patient outcome in colorectal carcinoma. Med Oncol 2018;35:104. [Crossref] [PubMed]
- Thiery JP. Epithelial-mesenchymal transitions in tumour progression. Nat Rev Cancer 2002;2:442-54. [Crossref] [PubMed]
- Yilmaz M, Christofori G. EMT, the cytoskeleton, and cancer cell invasion. Cancer Metastasis Rev 2009;28:15-33. [Crossref] [PubMed]
- Stenman UH, Alfthan H, Hotakainen K. Human chorionic gonadotropin in cancer. Clin Biochem 2004;37:549-61. [Crossref] [PubMed]
- Melzer C, Hass R, von der Ohe J, et al. The role of TGF-β and its crosstalk with RAC1/RAC1b signaling in breast and pancreas carcinoma. Cell Commun Signal 2017;15:19. [Crossref] [PubMed]
- Guasch G, Schober M, Pasolli HA, et al. Loss of TGFbeta signaling destabilizes homeostasis and promotes squamous cell carcinomas in stratified epithelia. Cancer Cell 2007;12:313-27. [Crossref] [PubMed]
- Heldin CH, Vanlandewijck M, Moustakas A. Regulation of EMT by TGFβ in cancer. FEBS Lett 2012;586:1959-70. [Crossref] [PubMed]
- Berndt S, Blacher S, Munaut C, et al. Hyperglycosylated human chorionic gonadotropin stimulates angiogenesis through TGF-β receptor activation. FASEB J 2013;27:1309-21. [Crossref] [PubMed]
- Wu W, Walker AM. Human chorionic gonadotropin beta (HCGbeta) down-regulates E-cadherin and promotes human prostate carcinoma cell migration and invasion. Cancer 2006;106:68-78. [Crossref] [PubMed]
- Carpelan-Holmström M, Louhimo J, Stenman UH, et al. CEA, CA 242, CA 19-9, CA 72-4 and hCGbeta in the diagnosis of recurrent colorectal cancer. Tumour Biol 2004;25:228-34. [Crossref] [PubMed]
- Louhimo J, Carpelan-Holmström M, Alfthan H, et al. Serum HCG beta, CA 72-4 and CEA are independent prognostic factors in colorectal cancer. Int J Cancer 2002;101:545-8. [Crossref] [PubMed]
- Birgisson H, Jirström K, Stenman UH. Serum concentrations of human chorionic gonadotropin beta and its association with survival in patients with colorectal cancer. Cancer Biomark 2012;11:173-81. [Crossref] [PubMed]
- Lundin M, Nordling S, Lundin J, et al. Tissue expression of human chorionic gonadotropin beta predicts outcome in colorectal cancer: a comparison with serum expression. Int J Cancer 2001;95:18-22. [Crossref] [PubMed]